The environment topography alters the way to multicellularity in Myxococcus xanthus
- PMID: 34433567
- PMCID: PMC8386931
- DOI: 10.1126/sciadv.abh2278
The environment topography alters the way to multicellularity in Myxococcus xanthus
Abstract
The social soil-dwelling bacterium Myxococcus xanthus can form multicellular structures, known as fruiting bodies. Experiments in homogeneous environments have shown that this process is affected by the physicochemical properties of the substrate, but they have largely neglected the role of complex topographies. We experimentally demonstrate that the topography alters single-cell motility and multicellular organization in M. xanthus In topographies realized by randomly placing silica particles over agar plates, we observe that the cells' interaction with particles drastically modifies the dynamics of cellular aggregation, leading to changes in the number, size, and shape of the fruiting bodies and even to arresting their formation in certain conditions. We further explore this type of cell-particle interaction in a computational model. These results provide fundamental insights into how the environment topography influences the emergence of complex multicellular structures from single cells, which is a fundamental problem of biological, ecological, and medical relevance.
Copyright © 2021 The Authors, some rights reserved; exclusive licensee American Association for the Advancement of Science. No claim to original U.S. Government Works. Distributed under a Creative Commons Attribution NonCommercial License 4.0 (CC BY-NC).
Figures
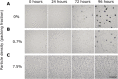
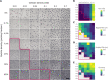
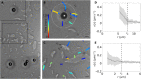
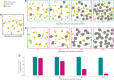
Similar articles
-
Unravelling a diversity of cellular structures and aggregation dynamics during the early development of Myxococcus xanthus.Biol Lett. 2024 Oct;20(10):20240360. doi: 10.1098/rsbl.2024.0360. Epub 2024 Oct 23. Biol Lett. 2024. PMID: 39439355 Free PMC article.
-
MglC, a Paralog of Myxococcus xanthus GTPase-Activating Protein MglB, Plays a Divergent Role in Motility Regulation.J Bacteriol. 2015 Nov 16;198(3):510-20. doi: 10.1128/JB.00548-15. Print 2016 Feb 1. J Bacteriol. 2015. PMID: 26574508 Free PMC article.
-
Cell-fate determination in Myxococcus xanthus development: Network dynamics and novel predictions.Dev Growth Differ. 2018 Feb;60(2):121-129. doi: 10.1111/dgd.12424. Epub 2018 Feb 13. Dev Growth Differ. 2018. PMID: 29441522
-
Molecular Mechanisms of Signaling in Myxococcus xanthus Development.J Mol Biol. 2016 Sep 25;428(19):3805-30. doi: 10.1016/j.jmb.2016.07.008. Epub 2016 Jul 16. J Mol Biol. 2016. PMID: 27430596 Review.
-
Cell behavior and cell-cell communication during fruiting body morphogenesis in Myxococcus xanthus.J Microbiol Methods. 2003 Dec;55(3):829-39. doi: 10.1016/j.mimet.2003.08.007. J Microbiol Methods. 2003. PMID: 14607429 Review.
Cited by
-
Active interface bulging in Bacillus subtilis swarms promotes self-assembly and biofilm formation.Proc Natl Acad Sci U S A. 2024 Jul 30;121(31):e2322025121. doi: 10.1073/pnas.2322025121. Epub 2024 Jul 25. Proc Natl Acad Sci U S A. 2024. PMID: 39052827 Free PMC article.
-
Mutation of self-binding sites in the promoter of the MrpC transcriptional regulator leads to asynchronous Myxococcus xanthus development.Front Microbiol. 2023 Nov 23;14:1293966. doi: 10.3389/fmicb.2023.1293966. eCollection 2023. Front Microbiol. 2023. PMID: 38075919 Free PMC article.
-
Myxococcus xanthus fruiting body morphology is important for spore recovery after exposure to environmental stress.Appl Environ Microbiol. 2024 Oct 23;90(10):e0166024. doi: 10.1128/aem.01660-24. Epub 2024 Oct 4. Appl Environ Microbiol. 2024. PMID: 39365039
-
Host Environment Shapes S. aureus Social Behavior as Revealed by Microscopy Pattern Formation and Dynamic Aggregation Analysis.Microorganisms. 2022 Feb 28;10(3):526. doi: 10.3390/microorganisms10030526. Microorganisms. 2022. PMID: 35336102 Free PMC article.
-
Unravelling a diversity of cellular structures and aggregation dynamics during the early development of Myxococcus xanthus.Biol Lett. 2024 Oct;20(10):20240360. doi: 10.1098/rsbl.2024.0360. Epub 2024 Oct 23. Biol Lett. 2024. PMID: 39439355 Free PMC article.
References
-
- D. Nishiguchi, Order and Fluctuations in Collective Dynamics of Swimming Bacteria: Experimental Exploration of Active Matter Physics (Springer Nature, 2020).