Review: Insights on Current FDA-Approved Monoclonal Antibodies Against Ebola Virus Infection
- PMID: 34526994
- PMCID: PMC8435780
- DOI: 10.3389/fimmu.2021.721328
Review: Insights on Current FDA-Approved Monoclonal Antibodies Against Ebola Virus Infection
Abstract
The unprecedented 2013-2016 West Africa Ebola outbreak accelerated several medical countermeasures (MCMs) against Ebola virus disease (EVD). Several investigational products (IPs) were used throughout the outbreak but were not conclusive for efficacy results. Only the Randomized Controlled Trial (RCT) on ZMapp was promising but inconclusive. More recently, during the second-largest Ebola outbreak in North Kivu and Ituri provinces, Democratic Republic of the Congo (DRC), four IPs, including one small molecule (Remdesivir), two monoclonal antibody (mAb) cocktails (ZMapp and REGN-EB3) and a single mAb (mAb114), were evaluated in an RCT, the Pamoja Tulinde Maisha (PALM) study. Two products (REGN-EB3 and mAb114) demonstrated efficacy as compared to the control arm, ZMapp. There were remarkably few side effects recorded in the trial. The FDA approved both medications in this scientifically sound study, marking a watershed moment in the field of EVD therapy. These products can be produced relatively inexpensively and can be stockpiled. The administration of mAbs in EVD patients appears to be safe and effective, while several critical knowledge gaps remain; the impact of early administration of Ebola-specific mAbs on developing a robust immune response for future Ebola virus exposure is unknown. The viral mutation escape, leading to resistance, presents a potential limitation for single mAb therapy; further improvements need to be explored. Understanding the contribution of Fc-mediated antibody functions such as antibody-dependent cellular cytotoxicity (ADCC) of those approved mAbs is still critical. The potential merit of combination therapy and post-exposure prophylaxis (PEP) need to be demonstrated. Furthermore, the PALM trial has accounted for 30% of mortality despite the administration of specific treatments. The putative role of EBOV soluble Glycoprotein (sGP) as a decoy to the immune system, the virus persistence, and relapses might be investigated for treatment failure. The development of pan-filovirus or pan-species mAbs remains essential for protection. The interaction between FDA-approved mAbs and vaccines remains unclear and needs to be investigated. In this review, we summarize the efficacy and safety results of the PALM study and review current research questions for the further development of mAbs in pre-exposure or emergency post-exposure use.
Keywords: Ebola virus; antibodies; filovirus; monoclonal; therapeutics.
Copyright © 2021 Tshiani Mbaya, Mukumbayi and Mulangu.
Conflict of interest statement
OTM is employed by Leidos Biomedical Research. SM is employed by Ridgeback Biotherapeutics, and is listed as inventor on the patent application for mAb 114, US Application No.62/087, 087 (PCT Application No. PCT/US2015/060733) related to anti-Ebola virus antibodies and their use. The remaining authors declare that the research was conducted in the absence of any commercial or financial relationships that could be construed as a potential conflict of interest.
Figures
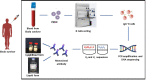
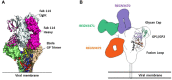
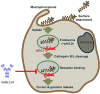
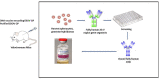
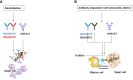
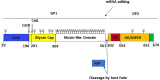
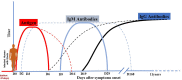
Similar articles
-
A Randomized, Controlled Trial of Ebola Virus Disease Therapeutics.N Engl J Med. 2019 Dec 12;381(24):2293-2303. doi: 10.1056/NEJMoa1910993. Epub 2019 Nov 27. N Engl J Med. 2019. PMID: 31774950 Free PMC article. Clinical Trial.
-
Medical countermeasures during the 2018 Ebola virus disease outbreak in the North Kivu and Ituri Provinces of the Democratic Republic of the Congo: a rapid genomic assessment.Lancet Infect Dis. 2019 Jun;19(6):648-657. doi: 10.1016/S1473-3099(19)30118-5. Epub 2019 Apr 15. Lancet Infect Dis. 2019. PMID: 31000464
-
Characterisation of infectious Ebola virus from the ongoing outbreak to guide response activities in the Democratic Republic of the Congo: a phylogenetic and in vitro analysis.Lancet Infect Dis. 2019 Sep;19(9):1023-1032. doi: 10.1016/S1473-3099(19)30291-9. Epub 2019 Jul 9. Lancet Infect Dis. 2019. PMID: 31300330 Free PMC article.
-
REGN-EB3: First Approval.Drugs. 2021 Jan;81(1):175-178. doi: 10.1007/s40265-020-01452-3. Drugs. 2021. PMID: 33432551 Free PMC article. Review.
-
Therapeutics development for Ebola virus disease: A recent scenario.Curr Opin Pharmacol. 2021 Oct;60:208-215. doi: 10.1016/j.coph.2021.07.020. Epub 2021 Aug 28. Curr Opin Pharmacol. 2021. PMID: 34464933 Review.
Cited by
-
Virology-The next fifty years.Cell. 2024 Sep 19;187(19):5128-5145. doi: 10.1016/j.cell.2024.07.025. Cell. 2024. PMID: 39303682
-
Ebola virus inclusion bodies are liquid organelles whose formation is facilitated by nucleoprotein oligomerization.Emerg Microbes Infect. 2023 Dec;12(2):2223727. doi: 10.1080/22221751.2023.2223727. Emerg Microbes Infect. 2023. PMID: 37306660 Free PMC article.
-
Reversion of Ebolavirus Disease from a Single Intramuscular Injection of a Pan-Ebolavirus Immunotherapeutic.Pathogens. 2022 Jun 7;11(6):655. doi: 10.3390/pathogens11060655. Pathogens. 2022. PMID: 35745509 Free PMC article.
-
A novel in vitro system of supported planar endosomal membranes (SPEMs) reveals an enhancing role for cathepsin B in the final stage of Ebola virus fusion and entry.Microbiol Spectr. 2023 Sep 20;11(5):e0190823. doi: 10.1128/spectrum.01908-23. Online ahead of print. Microbiol Spectr. 2023. PMID: 37728342 Free PMC article.
-
AAV-monoclonal antibody expression protects mice from Ebola virus without impeding the endogenous antibody response to heterologous challenge.Mol Ther Methods Clin Dev. 2022 Aug 12;26:505-518. doi: 10.1016/j.omtm.2022.08.003. eCollection 2022 Sep 8. Mol Ther Methods Clin Dev. 2022. PMID: 36092367 Free PMC article.
References
-
- CDC . History of Ebola Virus Disease (EVD) Outbreaks Error Processing SSI File (2021). Available at: https://www.cdc.gov/vhf/ebola/history/chronology.html.
Publication types
MeSH terms
Substances
LinkOut - more resources
Full Text Sources
Medical
Miscellaneous