The response of a boreal deep-sea sponge holobiont to acute thermal stress
- PMID: 28533520
- PMCID: PMC5440399
- DOI: 10.1038/s41598-017-01091-x
The response of a boreal deep-sea sponge holobiont to acute thermal stress
Abstract
Effects of elevated seawater temperatures on deep-water benthos has been poorly studied, despite reports of increased seawater temperature (up to 4 °C over 24 hrs) coinciding with mass mortality events of the sponge Geodia barretti at Tisler Reef, Norway. While the mechanisms driving these mortality events are unclear, manipulative laboratory experiments were conducted to quantify the effects of elevated temperature (up to 5 °C, above ambient levels) on the ecophysiology (respiration rate, nutrient uptake, cellular integrity and sponge microbiome) of G. barretti. No visible signs of stress (tissue necrosis or discolouration) were evident across experimental treatments; however, significant interactive effects of time and treatment on respiration, nutrient production and cellular stress were detected. Respiration rates and nitrogen effluxes doubled in responses to elevated temperatures (11 °C & 12 °C) compared to control temperatures (7 °C). Cellular stress, as measured through lysosomal destabilisation, was 2-5 times higher at elevated temperatures than for control temperatures. However, the microbiome of G. barretti remained stable throughout the experiment, irrespective of temperature treatment. Mortality was not evident and respiration rates returned to pre-experimental levels during recovery. These results suggest other environmental processes, either alone or in combination with elevated temperature, contributed to the mortality of G. barretti at Tisler reef.
Conflict of interest statement
The authors declare that they have no competing interests.
Figures
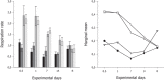
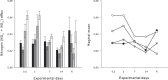
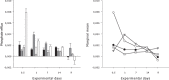
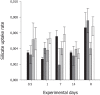
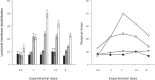
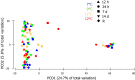
Similar articles
-
Group B streptococcus colonization in pregnancy and neonatal outcomes: a three-year monocentric retrospective study during and after the COVID-19 pandemic.Ital J Pediatr. 2024 Sep 13;50(1):175. doi: 10.1186/s13052-024-01738-2. Ital J Pediatr. 2024. PMID: 39267078 Free PMC article.
-
RETRACTED: Hydroxychloroquine and azithromycin as a treatment of COVID-19: results of an open-label non-randomized clinical trial.Int J Antimicrob Agents. 2020 Jul;56(1):105949. doi: 10.1016/j.ijantimicag.2020.105949. Epub 2020 Mar 20. Int J Antimicrob Agents. 2020. Retraction in: Int J Antimicrob Agents. 2025 Jan;65(1):107416. doi: 10.1016/j.ijantimicag.2024.107416. PMID: 32205204 Free PMC article. Retracted. Clinical Trial.
-
Interventions to increase patient and family involvement in escalation of care for acute life-threatening illness in community health and hospital settings.Cochrane Database Syst Rev. 2020 Dec 8;12(12):CD012829. doi: 10.1002/14651858.CD012829.pub2. Cochrane Database Syst Rev. 2020. PMID: 33285618 Free PMC article.
-
Depressing time: Waiting, melancholia, and the psychoanalytic practice of care.In: Kirtsoglou E, Simpson B, editors. The Time of Anthropology: Studies of Contemporary Chronopolitics. Abingdon: Routledge; 2020. Chapter 5. In: Kirtsoglou E, Simpson B, editors. The Time of Anthropology: Studies of Contemporary Chronopolitics. Abingdon: Routledge; 2020. Chapter 5. PMID: 36137063 Free Books & Documents. Review.
-
Effect of testing for cancer on cancer- or venous thromboembolism (VTE)-related mortality and morbidity in people with unprovoked VTE.Cochrane Database Syst Rev. 2021 Oct 1;10(10):CD010837. doi: 10.1002/14651858.CD010837.pub5. Cochrane Database Syst Rev. 2021. PMID: 34597414 Free PMC article. Review.
Cited by
-
Comparative physiology reveals heat stress disrupts acid-base homeostasis independent of symbiotic state in the model cnidarian Exaiptasia diaphana.J Exp Biol. 2024 Feb 15;227(4):jeb246222. doi: 10.1242/jeb.246222. Epub 2024 Feb 22. J Exp Biol. 2024. PMID: 38269486 Free PMC article.
-
Oxygen consumption rate of flatworms under the influence of wake- and sleep-promoting neurotransmitters.J Exp Zool A Ecol Integr Physiol. 2024 Dec;341(10):1130-1136. doi: 10.1002/jez.2828. Epub 2024 May 27. J Exp Zool A Ecol Integr Physiol. 2024. PMID: 38801005 Free PMC article.
-
Short-Term Exposure to High-Temperature Water Causes a Shift in the Microbiome of the Common Aquarium Sponge Lendenfeldia chondrodes.Microb Ecol. 2021 Jan;81(1):213-222. doi: 10.1007/s00248-020-01556-z. Epub 2020 Aug 7. Microb Ecol. 2021. PMID: 32767091 Free PMC article.
-
Experimental temperatures shape host microbiome diversity and composition.Glob Chang Biol. 2023 Jan;29(1):41-56. doi: 10.1111/gcb.16429. Epub 2022 Oct 17. Glob Chang Biol. 2023. PMID: 36251487 Free PMC article.
-
The Effects of Sampling and Storage Conditions on the Metabolite Profile of the Marine Sponge Geodia barretti.Front Chem. 2021 May 10;9:662659. doi: 10.3389/fchem.2021.662659. eCollection 2021. Front Chem. 2021. PMID: 34041223 Free PMC article.
References
Publication types
MeSH terms
LinkOut - more resources
Full Text Sources
Other Literature Sources