The Top 10 fungal pathogens in molecular plant pathology
- PMID: 22471698
- PMCID: PMC6638784
- DOI: 10.1111/j.1364-3703.2011.00783.x
The Top 10 fungal pathogens in molecular plant pathology
Erratum in
- Mol Plant Pathol. 2012 Sep;13(7):804
Abstract
The aim of this review was to survey all fungal pathologists with an association with the journal Molecular Plant Pathology and ask them to nominate which fungal pathogens they would place in a 'Top 10' based on scientific/economic importance. The survey generated 495 votes from the international community, and resulted in the generation of a Top 10 fungal plant pathogen list for Molecular Plant Pathology. The Top 10 list includes, in rank order, (1) Magnaporthe oryzae; (2) Botrytis cinerea; (3) Puccinia spp.; (4) Fusarium graminearum; (5) Fusarium oxysporum; (6) Blumeria graminis; (7) Mycosphaerella graminicola; (8) Colletotrichum spp.; (9) Ustilago maydis; (10) Melampsora lini, with honourable mentions for fungi just missing out on the Top 10, including Phakopsora pachyrhizi and Rhizoctonia solani. This article presents a short resumé of each fungus in the Top 10 list and its importance, with the intent of initiating discussion and debate amongst the plant mycology community, as well as laying down a bench-mark. It will be interesting to see in future years how perceptions change and what fungi will comprise any future Top 10.
© 2012 THE AUTHORS. MOLECULAR PLANT PATHOLOGY © 2012 BSPP AND BLACKWELL PUBLISHING LTD.
Figures
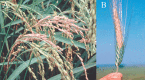
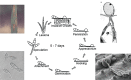
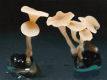
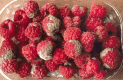
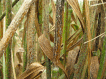
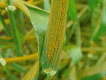
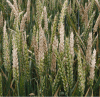
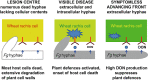
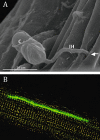
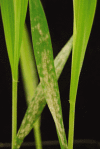
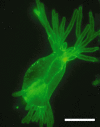
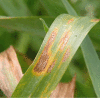
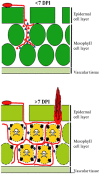
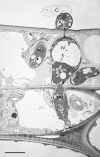
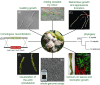
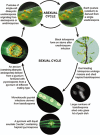
Similar articles
-
Top 10 plant pathogenic bacteria in molecular plant pathology.Mol Plant Pathol. 2012 Aug;13(6):614-29. doi: 10.1111/j.1364-3703.2012.00804.x. Epub 2012 Jun 5. Mol Plant Pathol. 2012. PMID: 22672649 Free PMC article. Review.
-
Top 10 plant viruses in molecular plant pathology.Mol Plant Pathol. 2011 Dec;12(9):938-54. doi: 10.1111/j.1364-3703.2011.00752.x. Epub 2011 Oct 21. Mol Plant Pathol. 2011. PMID: 22017770 Free PMC article. Review.
-
Cytochrome b gene structure and consequences for resistance to Qo inhibitor fungicides in plant pathogens.Pest Manag Sci. 2006 Jun;62(6):465-72. doi: 10.1002/ps.1236. Pest Manag Sci. 2006. PMID: 16688790
-
PHI-Nets: A Network Resource for Ascomycete Fungal Pathogens to Annotate and Identify Putative Virulence Interacting Proteins and siRNA Targets.Front Microbiol. 2019 Dec 6;10:2721. doi: 10.3389/fmicb.2019.02721. eCollection 2019. Front Microbiol. 2019. PMID: 31866958 Free PMC article.
-
Genes from mycoparasitic fungi as a source for improving plant resistance to fungal pathogens.Proc Natl Acad Sci U S A. 1998 Jul 7;95(14):7860-5. doi: 10.1073/pnas.95.14.7860. Proc Natl Acad Sci U S A. 1998. PMID: 9653105 Free PMC article.
Cited by
-
Cell biology of Zymoseptoria tritici: Pathogen cell organization and wheat infection.Fungal Genet Biol. 2015 Jun;79:17-23. doi: 10.1016/j.fgb.2015.04.002. Fungal Genet Biol. 2015. PMID: 26092785 Free PMC article. Review.
-
The peroxins BcPex8, BcPex10, and BcPex12 are required for the development and pathogenicity of Botrytis cinerea.Front Microbiol. 2022 Sep 6;13:962500. doi: 10.3389/fmicb.2022.962500. eCollection 2022. Front Microbiol. 2022. PMID: 36147853 Free PMC article.
-
The Plant Homeodomain Protein Clp1 Regulates Fungal Development, Virulence, and Autophagy Homeostasis in Magnaporthe oryzae.Microbiol Spectr. 2022 Oct 26;10(5):e0102122. doi: 10.1128/spectrum.01021-22. Epub 2022 Aug 29. Microbiol Spectr. 2022. PMID: 36036638 Free PMC article.
-
Jasmonate Signalling and Defence Responses in the Model Legume Medicago truncatula-A Focus on Responses to Fusarium Wilt Disease.Plants (Basel). 2016 Feb 5;5(1):11. doi: 10.3390/plants5010011. Plants (Basel). 2016. PMID: 27135231 Free PMC article.
-
Green Synthesis of Silver Nanoparticles with Hyssopus officinalis and Salvia officinalis Extracts, Their Properties, and Antifungal Activity on Fusarium spp.Plants (Basel). 2024 Jun 11;13(12):1611. doi: 10.3390/plants13121611. Plants (Basel). 2024. PMID: 38931043 Free PMC article.
References
-
- Agrios, G.N. (2005) Plant Pathology. St. Louis, MO: Academic Press.
-
- Alexander, N.J. , McCormick, S.P. , Waalwijk, C. , van der Lee, T. and Proctor, R.H. (2011) The genetic basis for 3‐ADON and 15‐ADON trichothecene chemotypes in Fusarium . Fungal Genet. Biol. 48, 485–495. - PubMed
-
- Amselem, J. , Cuomo, C.A. , van Kan, J.A.L. , Viaud, M. , Benito, E.P. , Couloux, A. , Coutinho, P.M. , de Vries, R.P. , Dyer, P.S. , Fillinger, S. , Fournier, E. , Gout, L. , Hahn, M. , Kohn, L. , Lapalu, N. , Plummer, K.M. , Pradier, J.‐M. , Quévillon, E. , Sharon, A. , Simon, A. , ten Have, A. , Tudzynski, B. , Tudzynski, P. , Wincker, P. , Andrew, M. , Anthouard, V. , Beever, R.E. , Beffa, R. , Benoit, I. , Bouzid, O. , Brault, B. , Chen, Z. , Choquer, M. , Collémare, J. , Cotton, P. , Danchin, E.G. , Da Silva, C. , Gautier, A. , Giraud, C. , Giraud, T. , Gonzalez, C. , Grossetete, S. , Güldener, U. , Henrissat, B. , Howlett, B. , Kodira, C. , Kretschmer, M. , Lappartient, A. , Leroch, M. , Levis, C. , Mauceli, E. , Neuvéglise, C. , Oeser, B. , Pearson, M. , Poulain, J. , Poussereau, N. , Quesneville, H. , Rascle, C. , Schumacher, J. , Ségurens, B. , Sexton, A. , Silva, E. , Sirven, C. , Soanes, D.M. , Talbot, N.J. , Templeton, M. , Yandava, C. , Yarden, O. , Zeng, Q. , Rollins, J.A. , Lebrun, M.‐H. and Dickman, M. (2011) Genomic analysis of the necrotrophic fungal pathogens Sclerotinia sclerotiorum and Botrytis cinerea . PLoS Genet. 7, e1002230. - PMC - PubMed
-
- Armstrong, G.M. and Armstrong, J.K. (1981) Formae speciales and races of Fusarium oxysporum causing wilt diseases In: Fusarium: Diseases, Biology and Taxonomy (Cook R., ed.), pp. 391–399. University Park, PA: Penn State University Press.
-
- von Arx, J.A. (1957) Die arten der gattung Colletotrichum Cda. Phytopathology Z. 29, 413–468.
Publication types
MeSH terms
Grants and funding
LinkOut - more resources
Full Text Sources
Other Literature Sources
Medical
Miscellaneous