Unicellular-multicellular evolutionary branching driven by resource limitations
- PMID: 35642429
- PMCID: PMC9156906
- DOI: 10.1098/rsif.2022.0018
Unicellular-multicellular evolutionary branching driven by resource limitations
Abstract
Multicellular life forms have evolved many times on our planet, suggesting that this is a common evolutionary innovation. Multiple advantages have been proposed for the emergence of multicellularity (MC). In this paper, we address the problem of how the first precondition for MC, namely 'stay together', might have occurred under spatially limited resources exploited by a population of unicellular agents. Using a minimal model of evolved cell-cell adhesion among growing and dividing cells that exploit a localized resource with a given size, we show that a transition occurs at a critical resource size separating a phase of evolved multicellular aggregates from a phase where unicellularity (UC) is favoured. The two phases are separated by an intermediate domain where both UC and MC can be selected by evolution. This model provides a minimal approach to the early stages that were required to transition from individuality to cohesive groups of cells associated with a physical cooperative effect: when resources are present only in a localized portion of the habitat, MC is a desirable property as it helps cells to keep close to the available local nutrients.
Keywords: cell adhesion; evolutionary preconditions; evolutionary transitions; multicellularity; statistical physics.
Figures
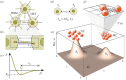
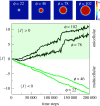
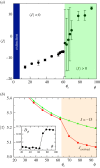
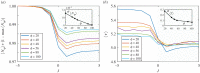
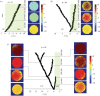
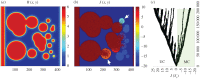
Similar articles
-
The Cost of Being Big: Local Competition, Importance of Dispersal, and Experimental Evolution of Reversal to Unicellularity.Am Nat. 2018 Dec;192(6):731-744. doi: 10.1086/700095. Epub 2018 Oct 24. Am Nat. 2018. PMID: 30444659
-
Evolution of multicellularity by collective integration of spatial information.Elife. 2020 Oct 16;9:e56349. doi: 10.7554/eLife.56349. Elife. 2020. PMID: 33064078 Free PMC article.
-
Evolutionary framework of the human interactome: Unicellular and multicellular giant clusters.Biosystems. 2019 Jul;181:82-87. doi: 10.1016/j.biosystems.2019.05.004. Epub 2019 May 8. Biosystems. 2019. PMID: 31077747
-
The origins of multicellularity and the early history of the genetic toolkit for animal development.Annu Rev Genet. 2008;42:235-51. doi: 10.1146/annurev.genet.42.110807.091513. Annu Rev Genet. 2008. PMID: 18983257 Review.
-
Diverse evolutionary paths to cell adhesion.Trends Cell Biol. 2010 Dec;20(12):734-42. doi: 10.1016/j.tcb.2010.08.002. Trends Cell Biol. 2010. PMID: 20817460 Free PMC article. Review.
Cited by
-
Group-selection via aggregative propagule-formation enables cooperative multicellularity in an individual based, spatial model.PLoS Comput Biol. 2024 May 7;20(5):e1012107. doi: 10.1371/journal.pcbi.1012107. eCollection 2024 May. PLoS Comput Biol. 2024. PMID: 38713735 Free PMC article.
-
Stability of ecologically scaffolded traits during evolutionary transitions in individuality.Nat Commun. 2024 Aug 3;15(1):6566. doi: 10.1038/s41467-024-50625-1. Nat Commun. 2024. PMID: 39095362 Free PMC article.
-
Morphological instability and roughening of growing 3D bacterial colonies.Proc Natl Acad Sci U S A. 2022 Oct 25;119(43):e2208019119. doi: 10.1073/pnas.2208019119. Epub 2022 Oct 18. Proc Natl Acad Sci U S A. 2022. PMID: 36256809 Free PMC article.
-
Temperature-Promoted Giant Unilamellar Vesicle (GUV) Aggregation: A Way of Multicellular Formation.Curr Issues Mol Biol. 2023 Apr 26;45(5):3757-3771. doi: 10.3390/cimb45050242. Curr Issues Mol Biol. 2023. PMID: 37232711 Free PMC article.
References
-
- Grosberg RK, Strathmann RR. 2007. The evolution of multicellularity: a minor major transition? Annu. Rev. Ecol. Evol. Syst. 38, 621-654. (10.1146/annurev.ecolsys.36.102403.114735) - DOI
-
- Knoll AH. 2011. The multiple origins of complex multicellularity. Annu. Rev. Earth Planet. Sci. 39, 217-239. (10.1146/annurev.earth.031208.100209) - DOI
-
- Ruiz-Trillo I, Nedelcu AM (eds). 2015. Evolutionary transitions to multicellular life: principles and mechanisms, vol. 2. Berlin, Germany: Springer.
-
- Bonner JT. 2001. First signals: the evolution of multicellular development. Princeton, NJ: Princeton University Press.
Publication types
MeSH terms
LinkOut - more resources
Full Text Sources