A c-di-GMP Signaling Cascade Controls Motility, Biofilm Formation, and Virulence in Burkholderia thailandensis
- PMID: 35323023
- PMCID: PMC9004398
- DOI: 10.1128/aem.02529-21
A c-di-GMP Signaling Cascade Controls Motility, Biofilm Formation, and Virulence in Burkholderia thailandensis
Abstract
As a key bacterial second messenger, cyclic di-GMP (c-di-GMP) regulates various physiological processes, such as motility, biofilm formation, and virulence. Cellular c-di-GMP levels are regulated by the opposing activities of diguanylate cyclases (DGCs) and phosphodiesterases (PDEs). Beyond that, the enzymatic activities of c-di-GMP metabolizing proteins are controlled by a variety of extracellular signals and intracellular physiological conditions. Here, we report that pdcA (BTH_II2363), pdcB (BTH_II2364), and pdcC (BTH_II2365) are cotranscribed in the same operon and are involved in a regulatory cascade controlling the cellular level of c-di-GMP in Burkholderia thailandensis. The GGDEF domain-containing protein PdcA was found to be a DGC that modulates biofilm formation, motility, and virulence in B. thailandensis. Moreover, the DGC activity of PdcA was inhibited by phosphorylated PdcC, a single-domain response regulator composed of only the phosphoryl-accepting REC domain. The phosphatase PdcB affects the function of PdcA by dephosphorylating PdcC. The observation that homologous operons of pdcABC are widespread among betaproteobacteria and gammaproteobacteria suggests a general mechanism by which the intracellular concentration of c-di-GMP is modulated to coordinate bacterial behavior and virulence. IMPORTANCE The transition from planktonic cells to biofilm cells is a successful strategy adopted by bacteria to survive in diverse environments, while the second messenger c-di-GMP plays an important role in this process. Cellular c-di-GMP levels are mainly controlled by modulating the activity of c-di-GMP-metabolizing proteins via the sensory domains adjacent to their enzymatic domains. However, in most cases how c-di-GMP-metabolizing enzymes are modulated by their sensory domains remains unclear. Here, we reveal a new c-di-GMP signaling cascade that regulates motility, biofilm formation, and virulence in B. thailandensis. While pdcA, pdcB, and pdcC constitute an operon, the phosphorylated PdcC binds the PAS sensory domain of PdcA to inhibit its DGC activity, with PdcB dephosphorylating PdcC to derepress the activity of PdcA. We also show this c-di-GMP regulatory model is widespread in the phylum Proteobacteria. Our study expands the current knowledge of how bacteria regulate intracellular c-di-GMP levels.
Keywords: Burkholderia thailandensis; biofilm; c-di-GMP; motility; virulence.
Conflict of interest statement
The authors declare no conflict of interest.
Figures
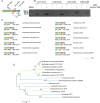
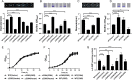
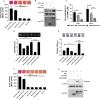
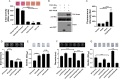
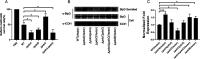
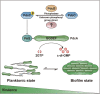
Similar articles
-
Enzymatically active and inactive phosphodiesterases and diguanylate cyclases are involved in regulation of Motility or sessility in Escherichia coli CFT073.mBio. 2012 Oct 9;3(5):e00307-12. doi: 10.1128/mBio.00307-12. mBio. 2012. PMID: 23047748 Free PMC article.
-
More than Enzymes That Make or Break Cyclic Di-GMP-Local Signaling in the Interactome of GGDEF/EAL Domain Proteins of Escherichia coli.mBio. 2017 Oct 10;8(5):e01639-17. doi: 10.1128/mBio.01639-17. mBio. 2017. PMID: 29018125 Free PMC article.
-
FlhF affects the subcellular clustering of WspR through HsbR in Pseudomonas aeruginosa.Appl Environ Microbiol. 2024 Jan 24;90(1):e0154823. doi: 10.1128/aem.01548-23. Epub 2023 Dec 19. Appl Environ Microbiol. 2024. PMID: 38112425 Free PMC article.
-
Targeting c-di-GMP Signaling, Biofilm Formation, and Bacterial Motility with Small Molecules.Methods Mol Biol. 2017;1657:419-430. doi: 10.1007/978-1-4939-7240-1_31. Methods Mol Biol. 2017. PMID: 28889311 Review.
-
The bacterial second messenger c-di-GMP: probing interactions with protein and RNA binding partners using cyclic dinucleotide analogs.Org Biomol Chem. 2012 Dec 14;10(46):9113-29. doi: 10.1039/c2ob26724a. Epub 2012 Oct 29. Org Biomol Chem. 2012. PMID: 23108253 Free PMC article. Review.
Cited by
-
Lsr2 acts as a cyclic di-GMP receptor that promotes keto-mycolic acid synthesis and biofilm formation in mycobacteria.Nat Commun. 2024 Jan 24;15(1):695. doi: 10.1038/s41467-024-44774-6. Nat Commun. 2024. PMID: 38267428 Free PMC article.
-
Identification of anti-pathogenic activity among in silico predicted small-molecule inhibitors of Pseudomonas aeruginosa LasR or nitric oxide reductase (NOR).Drug Target Insights. 2023 Sep 28;17:101-109. doi: 10.33393/dti.2023.2638. eCollection 2023 Jan-Dec. Drug Target Insights. 2023. PMID: 37811195 Free PMC article.
-
Biofilm Signaling, Composition and Regulation in Burkholderia pseudomallei.J Microbiol Biotechnol. 2023 Jan 28;33(1):15-27. doi: 10.4014/jmb.2207.07032. Epub 2022 Oct 17. J Microbiol Biotechnol. 2023. PMID: 36451302 Free PMC article. Review.
References
-
- Floyd KA, Lee CK, Xian W, Nametalla M, Valentine A, Crair B, Zhu S, Hughes HQ, Chlebek JL, Wu DC, Hwan Park J, Farhat AM, Lomba CJ, Ellison CK, Brun YV, Campos-Gomez J, Dalia AB, Liu J, Biais N, Wong GCL, Yildiz FH. 2020. c-di-GMP modulates type IV MSHA pilus retraction and surface attachment in Vibrio cholerae. Nat Commun 11:1549. 10.1038/s41467-020-15331-8. - DOI - PMC - PubMed
Publication types
MeSH terms
Substances
Supplementary concepts
LinkOut - more resources
Full Text Sources
Research Materials