Scientific Opinion addressing the state of the science on risk assessment of plant protection products for in-soil organisms
- PMID: 32625401
- PMCID: PMC7009882
- DOI: 10.2903/j.efsa.2017.4690
Scientific Opinion addressing the state of the science on risk assessment of plant protection products for in-soil organisms
Abstract
Following a request from EFSA, the Panel on Plant Protection Products and their Residues developed an opinion on the science behind the risk assessment of plant protection products for in-soil organisms. The current risk assessment scheme is reviewed, taking into account new regulatory frameworks and scientific developments. Proposals are made for specific protection goals for in-soil organisms being key drivers for relevant ecosystem services in agricultural landscapes such as nutrient cycling, soil structure, pest control and biodiversity. Considering the time-scales and biological processes related to the dispersal of the majority of in-soil organisms compared to terrestrial non-target arthropods living above soil, the Panel proposes that in-soil environmental risk assessments are made at in- and off-field scale considering field boundary levels. A new testing strategy which takes into account the relevant exposure routes for in-soil organisms and the potential direct and indirect effects is proposed. In order to address species recovery and long-term impacts of PPPs, the use of population models is also proposed.
Keywords: effects; in‐soil invertebrates; microorganisms; pesticides; protection goals; risk assessment.
© 2017 European Food Safety Authority. EFSA Journal published by John Wiley and Sons Ltd on behalf of European Food Safety Authority.
Figures
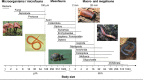
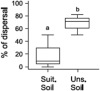
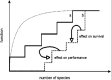
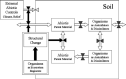
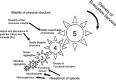
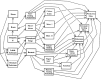
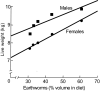
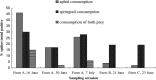
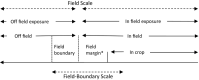
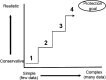
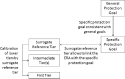
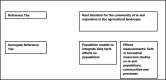
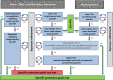
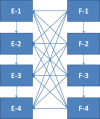
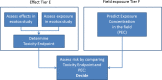
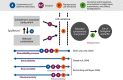
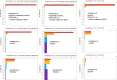
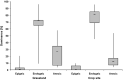
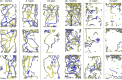
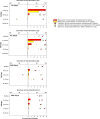
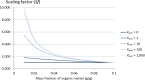
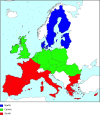
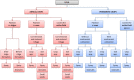
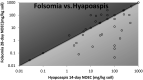
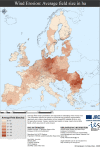
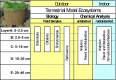
Similar articles
-
Environmental risk assessment of PPP application in European soils and potential ecosystem service losses considering impacts on non-target organisms.Ecotoxicol Environ Saf. 2023 Nov 1;266:115577. doi: 10.1016/j.ecoenv.2023.115577. Epub 2023 Oct 14. Ecotoxicol Environ Saf. 2023. PMID: 37839184
-
Scientific Opinion on the state of the science on pesticide risk assessment for amphibians and reptiles.EFSA J. 2018 Feb 23;16(2):e05125. doi: 10.2903/j.efsa.2018.5125. eCollection 2018 Feb. EFSA J. 2018. PMID: 32625798 Free PMC article.
-
Improved assessment of the impacts of plant protection products on certain soil ecosystem services requires better consideration of terrestrial microalgae and cyanobacteria.Environ Sci Pollut Res Int. 2025 Feb;32(6):2786-2793. doi: 10.1007/s11356-023-31198-w. Epub 2023 Nov 27. Environ Sci Pollut Res Int. 2025. PMID: 38010548 Review.
-
Arbuscular Mycorrhizal Fungi and the Need for a Meaningful Regulatory Plant Protection Product Testing Strategy.Environ Toxicol Chem. 2022 Aug;41(8):1808-1823. doi: 10.1002/etc.5400. Epub 2022 Jul 14. Environ Toxicol Chem. 2022. PMID: 35678214 Free PMC article. Review.
-
Wildlife ecotoxicology of plant protection products: knowns and unknowns about the impacts of currently used pesticides on terrestrial vertebrate biodiversity.Environ Sci Pollut Res Int. 2025 Feb;32(6):2893-2955. doi: 10.1007/s11356-024-33026-1. Epub 2024 Apr 19. Environ Sci Pollut Res Int. 2025. PMID: 38639904 Review.
Cited by
-
The Role of Source-Sink Dynamics in the Assessment of Risk to Nontarget Arthropods from the Use of Plant Protection Products.Environ Toxicol Chem. 2021 Oct;40(10):2667-2679. doi: 10.1002/etc.5137. Epub 2021 Aug 27. Environ Toxicol Chem. 2021. PMID: 34111321 Free PMC article. Review.
-
Avoidance Behaviour of Six Collembolan Species Shows Species-Specific Sensitivity-Impact of Ag NM300K.Nanomaterials (Basel). 2022 Sep 21;12(19):3276. doi: 10.3390/nano12193276. Nanomaterials (Basel). 2022. PMID: 36234404 Free PMC article.
-
Editorial: Microbial Ecotoxicology.Front Microbiol. 2020 Jun 26;11:1342. doi: 10.3389/fmicb.2020.01342. eCollection 2020. Front Microbiol. 2020. PMID: 32676059 Free PMC article. No abstract available.
-
Investigating the role of soil mesofauna abundance and biodiversity for organic matter breakdown in arable fields.Integr Environ Assess Manag. 2022 Sep;18(5):1423-1433. doi: 10.1002/ieam.4563. Epub 2021 Dec 21. Integr Environ Assess Manag. 2022. PMID: 34878731 Free PMC article.
-
Statement of the Scientific Panel on Plant Protection Products and their Residues (PPR Panel) on the design and conduct of groundwater monitoring studies supporting groundwater exposure assessments of pesticides.EFSA J. 2023 May 15;21(5):e07990. doi: 10.2903/j.efsa.2023.7990. eCollection 2023 May. EFSA J. 2023. PMID: 37197560 Free PMC article.
References
-
- Abd‐Alla M, Omar S and Karanxha S, 2000. The impact of pesticides on arbuscular mycorrhizal and nitrogen‐fixing symbioses in legumes. Applied Soil Ecology, 14, 191–200.
-
- Agerer R, 2006. Fungal relationships and structural identity of their ectomycorrhizae. Mycological Progress, 5, 67–107.
-
- Aguilar‐Trigueros C, Powell J, Anderson I, Antonovics J and Rillig M, 2014. Ecological understanding of root‐infecting fungi using trait‐based approaches. Trends in Plant Science, 19, 432–438. - PubMed
-
- Aislabie J and Deslippe J, 2013. Soil microbes and their contribution to soil services. In: Ecosystem services in New Zealand: conditions and trends. Manaaki Whenua Press, Lincoln, New Zealand, 143–161.
-
- Akhurst R and Smith K, 2002. Regulation and safety. In: Gaugler R (ed). Entomopathogenic nematology. CABI Publishing, Oxford. pp. 311–332.
LinkOut - more resources
Full Text Sources