Cathepsin S-cleavable, multi-block HPMA copolymers for improved SPECT/CT imaging of pancreatic cancer
- PMID: 27372424
- PMCID: PMC5018995
- DOI: 10.1016/j.biomaterials.2016.05.036
Cathepsin S-cleavable, multi-block HPMA copolymers for improved SPECT/CT imaging of pancreatic cancer
Abstract
This work continues our efforts to improve the diagnostic and radiotherapeutic effectiveness of nanomedicine platforms by developing approaches to reduce the non-target accumulation of these agents. Herein, we developed multi-block HPMA copolymers with backbones that are susceptible to cleavage by cathepsin S, a protease that is abundantly expressed in tissues of the mononuclear phagocyte system (MPS). Specifically, a bis-thiol terminated HPMA telechelic copolymer containing 1,4,7,10-tetraazacyclododecane-1,4,7,10-tetraacetic acid (DOTA) was synthesized by reversible addition-fragmentation chain transfer (RAFT) polymerization. Three maleimide modified linkers with different sequences, including cathepsin S degradable oligopeptide, scramble oligopeptide and oligo ethylene glycol, were subsequently synthesized and used for the extension of the HPMA copolymers by thiol-maleimide click chemistry. All multi-block HPMA copolymers could be labeled by (177)Lu with high labeling efficiency and exhibited high serum stability. In vitro cleavage studies demonstrated highly selective and efficient cathepsin S mediated cleavage of the cathepsin S-susceptible multi-block HPMA copolymer. A modified multi-block HPMA copolymer series capable of Förster Resonance Energy Transfer (FRET) was utilized to investigate the rate of cleavage of the multi-block HPMA copolymers in monocyte-derived macrophages. Confocal imaging and flow cytometry studies revealed substantially higher rates of cleavage for the multi-block HPMA copolymers containing the cathepsin S-susceptible linker. The efficacy of the cathepsin S-cleavable multi-block HPMA copolymer was further examined using an in vivo model of pancreatic ductal adenocarcinoma. Based on the biodistribution and SPECT/CT studies, the copolymer extended with the cathepsin S susceptible linker exhibited significantly faster clearance and lower non-target retention without compromising tumor targeting. Overall, these results indicate that exploitation of the cathepsin S activity in MPS tissues can be utilized to substantially lower non-target accumulation, suggesting this is a promising approach for the development of diagnostic and radiotherapeutic nanomedicine platforms.
Keywords: Cathepsin S; FRET imaging; HPMA; Mononuclear phagocyte system; Pancreatic cancer; SPECT/CT imaging.
Copyright © 2016 Elsevier Ltd. All rights reserved.
Figures
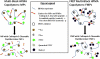
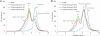
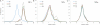
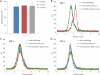
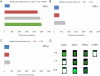
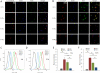
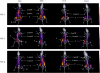
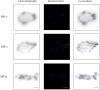
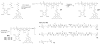
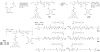
Similar articles
-
Investigation into the Biological Impact of Block Size on Cathepsin S-Degradable HPMA Copolymers.Mol Pharm. 2017 May 1;14(5):1405-1417. doi: 10.1021/acs.molpharmaceut.6b01038. Epub 2017 Mar 21. Mol Pharm. 2017. PMID: 28263073 Free PMC article.
-
The influence of linker length on the properties of cathepsin S cleavable (177)Lu-labeled HPMA copolymers for pancreatic cancer imaging.Biomaterials. 2014 Jul;35(22):5760-70. doi: 10.1016/j.biomaterials.2014.03.056. Epub 2014 Apr 19. Biomaterials. 2014. PMID: 24755528 Free PMC article.
-
177Lu-labeled HPMA copolymers utilizing cathepsin B and S cleavable linkers: synthesis, characterization and preliminary in vivo investigation in a pancreatic cancer model.Nucl Med Biol. 2013 Jul;40(5):606-17. doi: 10.1016/j.nucmedbio.2013.01.011. Epub 2013 Apr 24. Nucl Med Biol. 2013. PMID: 23622691 Free PMC article.
-
Gold nanorod-mediated hyperthermia enhances the efficacy of HPMA copolymer-90Y conjugates in treatment of prostate tumors.Nucl Med Biol. 2014 Mar;41(3):282-9. doi: 10.1016/j.nucmedbio.2013.12.002. Epub 2013 Dec 10. Nucl Med Biol. 2014. PMID: 24461626 Free PMC article.
-
Molecular imaging of HPMA copolymers: visualizing drug delivery in cell, mouse and man.Adv Drug Deliv Rev. 2010 Feb 17;62(2):246-57. doi: 10.1016/j.addr.2009.12.007. Epub 2010 Jan 7. Adv Drug Deliv Rev. 2010. PMID: 20060431 Review.
Cited by
-
Protease-triggered bioresponsive drug delivery for the targeted theranostics of malignancy.Acta Pharm Sin B. 2021 Aug;11(8):2220-2242. doi: 10.1016/j.apsb.2021.01.017. Epub 2021 Jan 24. Acta Pharm Sin B. 2021. PMID: 34522585 Free PMC article. Review.
-
Molecular Imaging of Hydrolytic Enzymes Using PET and SPECT.Mol Imaging. 2017 Jan-Dec;16:1536012117717852. doi: 10.1177/1536012117717852. Mol Imaging. 2017. PMID: 28927325 Free PMC article. Review.
-
Investigation into the Biological Impact of Block Size on Cathepsin S-Degradable HPMA Copolymers.Mol Pharm. 2017 May 1;14(5):1405-1417. doi: 10.1021/acs.molpharmaceut.6b01038. Epub 2017 Mar 21. Mol Pharm. 2017. PMID: 28263073 Free PMC article.
-
The Development of a Macromolecular Analgesic for Arthritic Pain.Mol Pharm. 2019 Mar 4;16(3):1234-1244. doi: 10.1021/acs.molpharmaceut.8b01197. Epub 2019 Feb 14. Mol Pharm. 2019. PMID: 30702897 Free PMC article.
-
Covalent nano delivery systems for selective imaging and treatment of brain tumors.Adv Drug Deliv Rev. 2017 Apr;113:177-200. doi: 10.1016/j.addr.2017.06.002. Epub 2017 Jun 10. Adv Drug Deliv Rev. 2017. PMID: 28606739 Free PMC article. Review.
References
-
- Siegel RL, Miller KD, Jemal A. Cancer statistics, 2015. CA: a cancer journal for clinicians. 2015;65:5–29. - PubMed
-
- Hanada K, Okazaki A, Hirano N, Izumi Y, Teraoka Y, Ikemoto J, et al. Diagnostic strategies for early pancreatic cancer. Journal of gastroenterology. 2015;50:147–54. - PubMed
-
- Fokas E, O'Neill E, Gordon-Weeks A, Mukherjee S, McKenna WG, Muschel RJ. Pancreatic ductal adenocarcinoma: From genetics to biology to radiobiology to oncoimmunology and all the way back to the clinic. Biochimica et Biophysica Acta (BBA)-Reviews on Cancer. 2015;1855:61–82. - PubMed
Publication types
MeSH terms
Substances
Grants and funding
LinkOut - more resources
Full Text Sources
Other Literature Sources
Medical
Miscellaneous