Dominant β-catenin mutations cause intellectual disability with recognizable syndromic features
- PMID: 24614104
- PMCID: PMC3973091
- DOI: 10.1172/JCI70372
Dominant β-catenin mutations cause intellectual disability with recognizable syndromic features
Abstract
The recent identification of multiple dominant mutations in the gene encoding β-catenin in both humans and mice has enabled exploration of the molecular and cellular basis of β-catenin function in cognitive impairment. In humans, β-catenin mutations that cause a spectrum of neurodevelopmental disorders have been identified. We identified de novo β-catenin mutations in patients with intellectual disability, carefully characterized their phenotypes, and were able to define a recognizable intellectual disability syndrome. In parallel, characterization of a chemically mutagenized mouse line that displays features similar to those of human patients with β-catenin mutations enabled us to investigate the consequences of β-catenin dysfunction through development and into adulthood. The mouse mutant, designated batface (Bfc), carries a Thr653Lys substitution in the C-terminal armadillo repeat of β-catenin and displayed a reduced affinity for membrane-associated cadherins. In association with this decreased cadherin interaction, we found that the mutation results in decreased intrahemispheric connections, with deficits in dendritic branching, long-term potentiation, and cognitive function. Our study provides in vivo evidence that dominant mutations in β-catenin underlie losses in its adhesion-related functions, which leads to severe consequences, including intellectual disability, childhood hypotonia, progressive spasticity of lower limbs, and abnormal craniofacial features in adults.
Figures
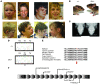
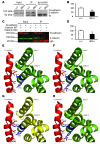
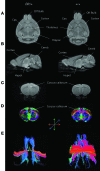
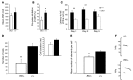
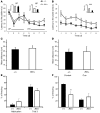
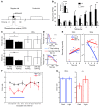
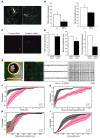
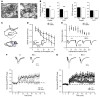
Similar articles
-
De novo mutations in beta-catenin (CTNNB1) appear to be a frequent cause of intellectual disability: expanding the mutational and clinical spectrum.Hum Genet. 2015 Jan;134(1):97-109. doi: 10.1007/s00439-014-1498-1. Epub 2014 Oct 19. Hum Genet. 2015. PMID: 25326669
-
Disruption of PHF21A causes syndromic intellectual disability with craniofacial anomalies, epilepsy, hypotonia, and neurobehavioral problems including autism.Mol Autism. 2019 Oct 22;10:35. doi: 10.1186/s13229-019-0286-0. eCollection 2019. Mol Autism. 2019. PMID: 31649809 Free PMC article.
-
A new intellectual disability syndrome caused by CTNNB1 haploinsufficiency.Am J Med Genet A. 2014 Jun;164A(6):1571-5. doi: 10.1002/ajmg.a.36484. Epub 2014 Mar 25. Am J Med Genet A. 2014. PMID: 24668549
-
Mechanistic insights from structural studies of beta-catenin and its binding partners.J Cell Sci. 2007 Oct 1;120(Pt 19):3337-44. doi: 10.1242/jcs.013771. J Cell Sci. 2007. PMID: 17881495 Review.
-
The KAT6B-related disorders genitopatellar syndrome and Ohdo/SBBYS syndrome have distinct clinical features reflecting distinct molecular mechanisms.Hum Mutat. 2012 Nov;33(11):1520-5. doi: 10.1002/humu.22141. Epub 2012 Jul 12. Hum Mutat. 2012. PMID: 22715153 Free PMC article. Review.
Cited by
-
Genetic and clinical characteristics of 24 mainland Chinese patients with CTNNB1 loss-of-function variants.Mol Genet Genomic Med. 2022 Nov;10(11):e2067. doi: 10.1002/mgg3.2067. Epub 2022 Sep 24. Mol Genet Genomic Med. 2022. PMID: 36153650 Free PMC article.
-
Genomic and phenotypic characterization of 404 individuals with neurodevelopmental disorders caused by CTNNB1 variants.Genet Med. 2022 Nov;24(11):2351-2366. doi: 10.1016/j.gim.2022.08.006. Epub 2022 Sep 9. Genet Med. 2022. PMID: 36083290 Free PMC article.
-
Learning impairments and molecular changes in the brain caused by β-catenin loss.Hum Mol Genet. 2019 Sep 1;28(17):2965-2975. doi: 10.1093/hmg/ddz115. Hum Mol Genet. 2019. PMID: 31131404 Free PMC article.
-
De novo mutations in beta-catenin (CTNNB1) appear to be a frequent cause of intellectual disability: expanding the mutational and clinical spectrum.Hum Genet. 2015 Jan;134(1):97-109. doi: 10.1007/s00439-014-1498-1. Epub 2014 Oct 19. Hum Genet. 2015. PMID: 25326669
-
A de novo CTNNB1 nonsense mutation associated with syndromic atypical hyperekplexia, microcephaly and intellectual disability: a case report.BMC Neurol. 2016 Mar 12;16:35. doi: 10.1186/s12883-016-0554-y. BMC Neurol. 2016. PMID: 26968164 Free PMC article.
References
Publication types
MeSH terms
Substances
Grants and funding
LinkOut - more resources
Full Text Sources
Other Literature Sources
Medical
Molecular Biology Databases
Research Materials
Miscellaneous