Cyclic di-GMP modulates sessile-motile phenotypes and virulence in Dickeya oryzae via two PilZ domain receptors
- PMID: 35254732
- PMCID: PMC9104268
- DOI: 10.1111/mpp.13200
Cyclic di-GMP modulates sessile-motile phenotypes and virulence in Dickeya oryzae via two PilZ domain receptors
Abstract
Dickeya oryzae is a bacterial pathogen causing the severe rice stem rot disease in China and other rice-growing countries. We showed recently that the universal bacterial second messenger c-di-GMP plays an important role in modulation of bacterial motility and pathogenicity, but the mechanism of regulation remains unknown. In this study, bioinformatics analysis of the D. oryzae EC1 genome led to the identification of two proteins, YcgR and BcsA, both of which contain a conserved c-di-GMP receptor domain, known as the PilZ-domain. By deleting all the genes encoding c-di-GMP-degrading enzymes in D. oryzae EC1, the resultant mutant 7ΔPDE with high c-di-GMP levels became nonmotile, formed hyperbiofilm, and lost the ability to colonize and invade rice seeds. These phenotypes were partially reversed by deletion of ycgR in the mutant 7ΔPDE, whereas deletion of bcsA only reversed the hyperbiofilm phenotype of mutant 7ΔPDE. Significantly, double deletion of ycgR and bcsA in mutant 7ΔPDE rescued its motility, biofilm formation, and virulence to levels of wild-type EC1. In vitro biochemical experiments and in vivo phenotypic assays further validated that YcgR and BcsA proteins are the receptors for c-di-GMP, which together play a critical role in regulating the c-di-GMP-associated functionality. The findings from this study fill a gap in our understanding of how c-di-GMP modulates bacterial motility and biofilm formation, and provide useful clues for further elucidation of sophisticated virulence regulatory mechanisms in this important plant pathogen.
Keywords: Dickeya oryzae; PilZ domain receptor; c-di-GMP; sessile-motile transition; virulence.
© 2022 The Authors. Molecular Plant Pathology published by British Society for Plant Pathology and John Wiley & Sons Ltd.
Conflict of interest statement
Authors declare that there are no known conflicts of interest associated with this paper.
Figures
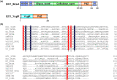
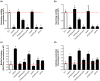
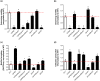
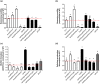
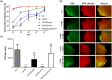
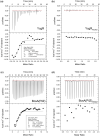
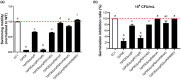
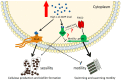
Similar articles
-
Systematic Analysis of c-di-GMP Signaling Mechanisms and Biological Functions in Dickeya zeae EC1.mBio. 2020 Dec 1;11(6):e02993-20. doi: 10.1128/mBio.02993-20. mBio. 2020. PMID: 33262261 Free PMC article.
-
The Xanthomonas oryzae pv. oryzae PilZ Domain Proteins Function Differentially in Cyclic di-GMP Binding and Regulation of Virulence and Motility.Appl Environ Microbiol. 2015 Jul;81(13):4358-67. doi: 10.1128/AEM.04044-14. Epub 2015 Apr 24. Appl Environ Microbiol. 2015. PMID: 25911481 Free PMC article.
-
The PilZ domain is a receptor for the second messenger c-di-GMP: the PilZ domain protein YcgR controls motility in enterobacteria.J Biol Chem. 2006 Oct 13;281(41):30310-4. doi: 10.1074/jbc.C600179200. Epub 2006 Aug 18. J Biol Chem. 2006. PMID: 16920715
-
Emerging paradigms for PilZ domain-mediated C-di-GMP signaling.Biochem Soc Trans. 2019 Feb 28;47(1):381-388. doi: 10.1042/BST20180543. Epub 2019 Feb 1. Biochem Soc Trans. 2019. PMID: 30710060 Review.
-
Identification of c-di-GMP Signaling Components in Xanthomonas oryzae and Their Orthologs in Xanthomonads Involved in Regulation of Bacterial Virulence Expression.Front Microbiol. 2019 Jul 11;10:1402. doi: 10.3389/fmicb.2019.01402. eCollection 2019. Front Microbiol. 2019. PMID: 31354637 Free PMC article. Review.
Cited by
-
Phylogenetic Analysis and Characterization of Diguanylate Cyclase and Phosphodiesterase in Planktonic Filamentous Cyanobacterium Arthrospira sp.Int J Mol Sci. 2023 Oct 16;24(20):15210. doi: 10.3390/ijms242015210. Int J Mol Sci. 2023. PMID: 37894891 Free PMC article.
-
A cyclic di-GMP-binding adaptor protein interacts with a N5-glutamine methyltransferase to regulate the pathogenesis in Xanthomonas citri subsp. citri.Mol Plant Pathol. 2024 Jul;25(7):e13496. doi: 10.1111/mpp.13496. Mol Plant Pathol. 2024. PMID: 39011828 Free PMC article.
-
Genome characterization of a uropathogenic Pseudomonas aeruginosa isolate PA_HN002 with cyclic di-GMP-dependent hyper-biofilm production.Front Cell Infect Microbiol. 2022 Aug 2;12:956445. doi: 10.3389/fcimb.2022.956445. eCollection 2022. Front Cell Infect Microbiol. 2022. PMID: 36004331 Free PMC article.
-
The integration host factor regulates multiple virulence pathways in bacterial pathogen Dickeya zeae MS2.Mol Plant Pathol. 2022 Oct;23(10):1487-1507. doi: 10.1111/mpp.13244. Epub 2022 Jul 12. Mol Plant Pathol. 2022. PMID: 35819797 Free PMC article.
-
Pseudomonas chlororaphis L5 and Enterobacter asburiae L95 biocontrol Dickeya soft rot diseases by quenching virulence factor modulating quorum sensing signal.Microb Biotechnol. 2023 Nov;16(11):2145-2160. doi: 10.1111/1751-7915.14351. Epub 2023 Oct 10. Microb Biotechnol. 2023. PMID: 37815509 Free PMC article.
References
-
- Amikam, D. & Galperin, M.Y. (2006) PilZ domain is part of the bacterial c‐di‐GMP binding protein. Bioinformatics, 22, 3–6. - PubMed
-
- Cheang, Q.W. , Xin, L. , Chea, R.Y.F. & Liang, Z.X. (2019) Emerging paradigms for PilZ domain‐mediated c‐di‐GMP signaling. Biochemical Society Transactions, 47, 381–388. - PubMed
Publication types
MeSH terms
Substances
LinkOut - more resources
Full Text Sources
Miscellaneous