Bacterial communities in carnivorous pitcher plants colonize and persist in inquiline mosquitoes
- PMID: 35172907
- PMCID: PMC8848819
- DOI: 10.1186/s42523-022-00164-1
Bacterial communities in carnivorous pitcher plants colonize and persist in inquiline mosquitoes
Abstract
Background: The leaves of carnivorous pitcher plants harbor diverse communities of inquiline species, including bacteria and larvae of the pitcher plant mosquito (Wyeomyia smithii), which aid the plant by processing captured prey. Despite the growing appreciation for this microecosystem as a tractable model in which to study food web dynamics and the moniker of W. smithii as a 'keystone predator', very little is known about microbiota acquisition and assembly in W. smithii mosquitoes or the impacts of W. smithii-microbiota interactions on mosquito and/or plant fitness.
Results: In this study, we used high throughput sequencing of bacterial 16S rRNA gene amplicons to characterize and compare microbiota diversity in field- and laboratory-derived W. smithii larvae. We then conducted controlled experiments in the laboratory to better understand the factors shaping microbiota acquisition and persistence across the W. smithii life cycle. Methods were also developed to produce axenic (microbiota-free) W. smithii larvae that can be selectively recolonized with one or more known bacterial species in order to study microbiota function. Our results support a dominant role for the pitcher environment in shaping microbiota diversity in W. smithii larvae, while also indicating that pitcher-associated microbiota can persist in and be dispersed by adult W. smithii mosquitoes. We also demonstrate the successful generation of axenic W. smithii larvae and report variable fitness outcomes in gnotobiotic larvae monocolonized by individual bacterial isolates derived from naturally occurring pitchers in the field.
Conclusions: This study provides the first information on microbiota acquisition and assembly in W. smithii mosquitoes. This study also provides the first evidence for successful microbiota manipulation in this species. Altogether, our results highlight the value of such methods for studying host-microbiota interactions and lay the foundation for future studies to understand how W. smithii-microbiota interactions shape the structure and stability of this important model ecosystem.
Keywords: Development; Life history; Microbiota diversity; Mosquito; Wyeomyia smithii.
© 2022. The Author(s).
Conflict of interest statement
The authors declare that they have no competing interests.
Figures
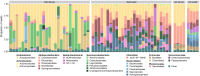
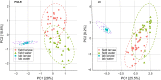
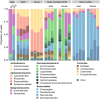
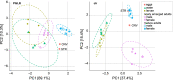
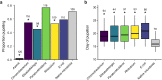
Similar articles
-
An inquiline mosquito modulates microbial diversity and function in an aquatic microecosystem.Mol Ecol. 2024 Apr;33(7):e17314. doi: 10.1111/mec.17314. Epub 2024 Mar 5. Mol Ecol. 2024. PMID: 38441172
-
A keystone predator controls bacterial diversity in the pitcher-plant (Sarracenia purpurea) microecosystem.Environ Microbiol. 2008 Sep;10(9):2257-66. doi: 10.1111/j.1462-2920.2008.01648.x. Epub 2008 May 11. Environ Microbiol. 2008. PMID: 18479443
-
Diverse microbial communities hosted by the model carnivorous pitcher plant Sarracenia purpurea: analysis of both bacterial and eukaryotic composition across distinct host plant populations.PeerJ. 2019 Feb 18;7:e6392. doi: 10.7717/peerj.6392. eCollection 2019. PeerJ. 2019. PMID: 30805246 Free PMC article.
-
Traps of carnivorous pitcher plants as a habitat: composition of the fluid, biodiversity and mutualistic activities.Ann Bot. 2011 Feb;107(2):181-94. doi: 10.1093/aob/mcq238. Epub 2010 Dec 15. Ann Bot. 2011. PMID: 21159782 Free PMC article. Review.
-
Naturally Occurring Microbiota Associated with Mosquito Breeding Habitats and Their Effects on Mosquito Larvae.Biomed Res Int. 2020 Dec 14;2020:4065315. doi: 10.1155/2020/4065315. eCollection 2020. Biomed Res Int. 2020. PMID: 33381553 Free PMC article. Review.
Cited by
-
An inquiline mosquito modulates microbial diversity and function in an aquatic microecosystem.Mol Ecol. 2024 Apr;33(7):e17314. doi: 10.1111/mec.17314. Epub 2024 Mar 5. Mol Ecol. 2024. PMID: 38441172
-
Interkingdom interactions shape the fungal microbiome of mosquitoes.Anim Microbiome. 2024 Mar 7;6(1):11. doi: 10.1186/s42523-024-00298-4. Anim Microbiome. 2024. PMID: 38454530 Free PMC article.
References
-
- Ellison AM, Gotelli NJ. Scaling in ecology with a model system. Princeton: University Press; 2021.
-
- Bradshaw WE, Creelman RA. Mutualism between the carnivorous purple pitcher plant and its inhabitants. Am Midl Nat. 1984;112:294.
-
- Butler JL, Gotelli NJ, Ellison AM. Linking the brown and green: nutrient transformation and fate in the Sarracenia microecosystem. Ecology. 2008;89:898–904. - PubMed
Grants and funding
LinkOut - more resources
Full Text Sources
Other Literature Sources