Nanogels: A novel approach in antimicrobial delivery systems and antimicrobial coatings
- PMID: 33898869
- PMCID: PMC8047124
- DOI: 10.1016/j.bioactmat.2021.03.004
Nanogels: A novel approach in antimicrobial delivery systems and antimicrobial coatings
Abstract
The implementation of nanotechnology to develop efficient antimicrobial systems has a significant impact on the prospects of the biomedical field. Nanogels are soft polymeric particles with an internally cross-linked structure, which behave as hydrogels and can be reversibly hydrated/dehydrated (swollen/shrunken) by the dispersing solvent and external stimuli. Their excellent properties, such as biocompatibility, colloidal stability, high water content, desirable mechanical properties, tunable chemical functionalities, and interior gel-like network for the incorporation of biomolecules, make them fascinating in the field of biological/biomedical applications. In this review, various approaches will be discussed and compared to the newly developed nanogel technology in terms of efficiency and applicability for determining their potential role in combating infections in the biomedical area including implant-associated infections.
Keywords: Antibacterial activity; Antifouling; Biocompatibility; Coatings; Nanogels.
© 2021 The Authors.
Conflict of interest statement
The authors declare the following conflict of interests: P. van Rijn also is co-founder, scientific advisor, and shareholder of BiomACS BV, a biomedical-oriented screening company.
Figures
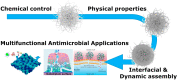
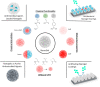
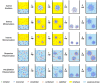
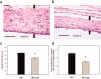
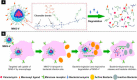
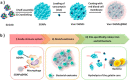
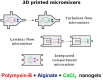
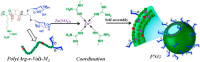
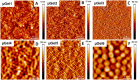
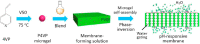
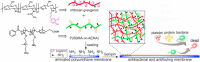
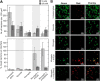
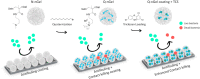
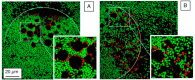
Similar articles
-
Highly Efficient Antimicrobial and Antifouling Surface Coatings with Triclosan-Loaded Nanogels.ACS Appl Mater Interfaces. 2020 Dec 30;12(52):57721-57731. doi: 10.1021/acsami.0c18172. Epub 2020 Dec 15. ACS Appl Mater Interfaces. 2020. PMID: 33320528 Free PMC article.
-
Polyglycerol-based hydrogels and nanogels: from synthesis to applications.Future Med Chem. 2021 Feb;13(4):419-438. doi: 10.4155/fmc-2020-0205. Epub 2021 Jan 6. Future Med Chem. 2021. PMID: 33403867 Review.
-
An insight of nanogels as novel drug delivery system with potential hybrid nanogel applications.J Biomater Sci Polym Ed. 2022 Feb;33(2):262-278. doi: 10.1080/09205063.2021.1982643. Epub 2021 Oct 10. J Biomater Sci Polym Ed. 2022. PMID: 34547214
-
Biomedicine Innovations and Its Nanohydrogel Classifications.Pharmaceutics. 2022 Dec 18;14(12):2839. doi: 10.3390/pharmaceutics14122839. Pharmaceutics. 2022. PMID: 36559335 Free PMC article. Review.
-
Chemical, physical, and biological stimuli-responsive nanogels for biomedical applications (mechanisms, concepts, and advancements): A review.Int J Biol Macromol. 2023 Jan 31;226:535-553. doi: 10.1016/j.ijbiomac.2022.12.076. Epub 2022 Dec 12. Int J Biol Macromol. 2023. PMID: 36521697 Review.
Cited by
-
Nanomaterial-Based Zinc Ion Interference Therapy to Combat Bacterial Infections.Front Immunol. 2022 Jun 30;13:899992. doi: 10.3389/fimmu.2022.899992. eCollection 2022. Front Immunol. 2022. PMID: 35844505 Free PMC article. Review.
-
Titanium or Biodegradable Osteosynthesis in Maxillofacial Surgery? In Vitro and In Vivo Performances.Polymers (Basel). 2022 Jul 7;14(14):2782. doi: 10.3390/polym14142782. Polymers (Basel). 2022. PMID: 35890557 Free PMC article. Review.
-
Transforming Medicine with Nanobiotechnology: Nanocarriers and Their Biomedical Applications.Pharmaceutics. 2024 Aug 23;16(9):1114. doi: 10.3390/pharmaceutics16091114. Pharmaceutics. 2024. PMID: 39339152 Free PMC article. Review.
-
Modern Herbal Nanogels: Formulation, Delivery Methods, and Applications.Gels. 2022 Feb 7;8(2):97. doi: 10.3390/gels8020097. Gels. 2022. PMID: 35200478 Free PMC article. Review.
-
3D-Printable Hierarchical Nanogel-GelMA Composite Hydrogel System.Polymers (Basel). 2021 Jul 29;13(15):2508. doi: 10.3390/polym13152508. Polymers (Basel). 2021. PMID: 34372111 Free PMC article.
References
-
- Rostad C.A., Wehrheim K., Kirklin J.K., Naftel D., Pruitt E., Hoffman T.M., L'Ecuyer T., Berkowitz K., Mahle W.T., Scheel J.N. Bacterial infections after pediatric heart transplantation: epidemiology, risk factors and outcomes. J. Heart Lung Transplant. 2017;36:996–1003. doi: 10.1016/j.healun.2017.05.009. - DOI - PubMed
-
- Shepshelovich D., Tau N., Green H., Rozen-Zvi B., Issaschar A., Falcone M., Coussement J., Zusman O., Manuel O., Mor E., Torre-Cisneros J., Yahav D. Immunosuppression reduction in liver and kidney transplant recipients with suspected bacterial infection: a multinational survey. Transpl. Infect. Dis. 2019;21:e13134. doi: 10.1111/tid.13134. - DOI - PubMed
Publication types
LinkOut - more resources
Full Text Sources
Other Literature Sources