Biosurfactants Produced by Phyllosphere-Colonizing Pseudomonads Impact Diesel Degradation but Not Colonization of Leaves of Gnotobiotic Arabidopsis thaliana
- PMID: 33608298
- PMCID: PMC8091026
- DOI: 10.1128/AEM.00091-21
Biosurfactants Produced by Phyllosphere-Colonizing Pseudomonads Impact Diesel Degradation but Not Colonization of Leaves of Gnotobiotic Arabidopsis thaliana
Abstract
Biosurfactant production is a common trait in leaf surface-colonizing bacteria that has been associated with increased survival and movement on leaves. At the same time, the ability to degrade aliphatics is common in biosurfactant-producing leaf colonizers. Pseudomonads are common leaf colonizers and have been recognized for their ability to produce biosurfactants and degrade aliphatic compounds. In this study, we investigated the role of biosurfactants in four non-plant-pathogenic Pseudomonas strains by performing a series of experiments to characterize their surfactant properties and their role during leaf colonization and diesel degradation. The biosurfactants produced were identified using mass spectrometry. Two strains produced viscosin-like biosurfactants, and the other two produced massetolide A-like biosurfactants, which aligned with the phylogenetic relatedness between the strains. To further investigate the role of surfactant production, random Tn5 transposon mutagenesis was performed to generate knockout mutants. The knockout mutants were compared to their respective wild types with regard to their ability to colonize gnotobiotic Arabidopsis thaliana and to degrade diesel or dodecane. It was not possible to detect negative effects during plant colonization in direct competition or individual colonization experiments. When grown on diesel, knockout mutants grew significantly slower than their respective wild types. When grown on dodecane, knockout mutants were less impacted than during growth on diesel. By adding isolated wild-type biosurfactants, it was possible to complement the growth of the knockout mutants.IMPORTANCE Many leaf-colonizing bacteria produce surfactants and are able to degrade aliphatic compounds; however, whether surfactant production provides a competitive advantage during leaf colonization is unclear. Furthermore, it is unclear if leaf colonizers take advantage of the aliphatic compounds that constitute the leaf cuticle and cuticular waxes. Here, we tested the effect of surfactant production on leaf colonization, and we demonstrate that the lack of surfactant production decreases the ability to degrade aliphatic compounds. This indicates that leaf surface-dwelling, surfactant-producing bacteria contribute to degradation of environmental hydrocarbons and may be able to utilize leaf surface waxes. This has implications for plant-microbe interactions and future studies.
Keywords: aliphatics; leaf cuticle; leaf wax; massetolide A; phyllosphere-inhabiting microbes; plant-microbe interactions; viscosin.
Copyright © 2021 American Society for Microbiology.
Figures
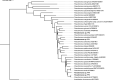
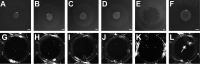
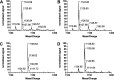
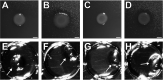
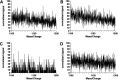
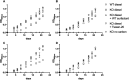
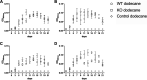
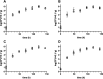
Similar articles
-
Utilisation of hydrocarbons and production of surfactants by bacteria isolated from plant leaf surfaces.FEMS Microbiol Lett. 2019 Mar 1;366(6):fnz061. doi: 10.1093/femsle/fnz061. FEMS Microbiol Lett. 2019. PMID: 30916756
-
Biosurfactant production by Pseudomonas strains isolated from floral nectar.J Appl Microbiol. 2015 Jun;118(6):1370-84. doi: 10.1111/jam.12799. Epub 2015 Apr 22. J Appl Microbiol. 2015. PMID: 25801599
-
Properties and potential application of efficient biosurfactant produced by Pseudomonas sp. KZ1 strain.J Environ Sci Health A Tox Hazard Subst Environ Eng. 2019;54(2):110-117. doi: 10.1080/10934529.2018.1530537. Epub 2019 Jan 7. J Environ Sci Health A Tox Hazard Subst Environ Eng. 2019. PMID: 30614383
-
Biosurfactants in agriculture.Appl Microbiol Biotechnol. 2013 Feb;97(3):1005-16. doi: 10.1007/s00253-012-4641-8. Epub 2013 Jan 3. Appl Microbiol Biotechnol. 2013. PMID: 23280539 Free PMC article. Review.
-
Genetic regulations of the biosynthesis of microbial surfactants: an overview.Biotechnol Genet Eng Rev. 2008;25:165-85. doi: 10.5661/bger-25-165. Biotechnol Genet Eng Rev. 2008. PMID: 21412355 Review.
Cited by
-
Hitching a Ride in the Phyllosphere: Surfactant Production of Pseudomonas spp. Causes Co-swarming of Pantoea eucalypti 299R.Microb Ecol. 2024 Apr 29;87(1):62. doi: 10.1007/s00248-024-02381-4. Microb Ecol. 2024. PMID: 38683223 Free PMC article.
-
Biosurfactant production by Bacillus cereus GX7 utilizing organic waste and its application in the remediation of hydrocarbon-contaminated environments.World J Microbiol Biotechnol. 2024 Oct 3;40(11):334. doi: 10.1007/s11274-024-04115-7. World J Microbiol Biotechnol. 2024. PMID: 39358641
-
An Nuclear Magnetic Resonance Fingerprint Matching Approach for the Identification and Structural Re-Evaluation of Pseudomonas Lipopeptides.Microbiol Spectr. 2022 Aug 31;10(4):e0126122. doi: 10.1128/spectrum.01261-22. Epub 2022 Jul 25. Microbiol Spectr. 2022. PMID: 35876524 Free PMC article. Review.
References
-
- Zeisler-Diehl VV, Barthlott W, Schreiber L. 2018. Plant cuticular waxes: composition, function, and interactions with microorganisms, p 1–16. In Wilkes H (ed), Hydrocarbons, oils and lipids: diversity, origin, chemistry and fate. Springer International Publishing, Cham, Switzerland.
-
- Yeats TH, Buda GJ, Wang Z, Chehanovsky N, Moyle LC, Jetter R, Schaffer AA, Rose JKC. 2012. The fruit cuticles of wild tomato species exhibit architectural and chemical diversity, providing a new model for studying the evolution of cuticle function. Plant J 69:655–666. doi:10.1111/j.1365-313X.2011.04820.x. - DOI - PMC - PubMed
MeSH terms
Substances
LinkOut - more resources
Full Text Sources
Other Literature Sources