Extracellular matrix dynamics in cell migration, invasion and tissue morphogenesis
- PMID: 31179622
- PMCID: PMC6658910
- DOI: 10.1111/iep.12329
Extracellular matrix dynamics in cell migration, invasion and tissue morphogenesis
Abstract
This review describes how direct visualization of the dynamic interactions of cells with different extracellular matrix microenvironments can provide novel insights into complex biological processes. Recent studies have moved characterization of cell migration and invasion from classical 2D culture systems into 1D and 3D model systems, revealing multiple differences in mechanisms of cell adhesion, migration and signalling-even though cells in 3D can still display prominent focal adhesions. Myosin II restrains cell migration speed in 2D culture but is often essential for effective 3D migration. 3D cell migration modes can switch between lamellipodial, lobopodial and/or amoeboid depending on the local matrix environment. For example, "nuclear piston" migration can be switched off by local proteolysis, and proteolytic invadopodia can be induced by a high density of fibrillar matrix. Particularly, complex remodelling of both extracellular matrix and tissues occurs during morphogenesis. Extracellular matrix supports self-assembly of embryonic tissues, but it must also be locally actively remodelled. For example, surprisingly focal remodelling of the basement membrane occurs during branching morphogenesis-numerous tiny perforations generated by proteolysis and actomyosin contractility produce a microscopically porous, flexible basement membrane meshwork for tissue expansion. Cells extend highly active blebs or protrusions towards the surrounding mesenchyme through these perforations. Concurrently, the entire basement membrane undergoes translocation in a direction opposite to bud expansion. Underlying this slowly moving 2D basement membrane translocation are highly dynamic individual cell movements. We conclude this review by describing a variety of exciting research opportunities for discovering novel insights into cell-matrix interactions.
Keywords: 3D culture; basement membrane remodelling; branching morphogenesis; cell migration; extracellular matrix; invasion.
© 2019 The Authors. International Journal of Experimental Pathology © 2019 International Journal of Experimental Pathology.
Conflict of interest statement
The authors declare no conflicts of interest.
Figures
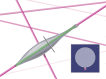
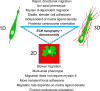
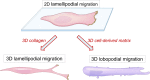
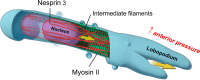
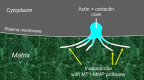
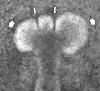
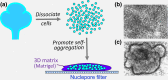
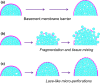
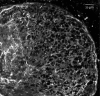
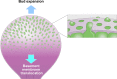
Similar articles
-
Mechanisms of 3D cell migration.Nat Rev Mol Cell Biol. 2019 Dec;20(12):738-752. doi: 10.1038/s41580-019-0172-9. Epub 2019 Oct 3. Nat Rev Mol Cell Biol. 2019. PMID: 31582855 Review.
-
The matrix environmental and cell mechanical properties regulate cell migration and contribute to the invasive phenotype of cancer cells.Rep Prog Phys. 2019 Jun;82(6):064602. doi: 10.1088/1361-6633/ab1628. Epub 2019 Apr 4. Rep Prog Phys. 2019. PMID: 30947151 Review.
-
Local and global dynamics of the basement membrane during branching morphogenesis require protease activity and actomyosin contractility.Dev Biol. 2014 Oct 15;394(2):197-205. doi: 10.1016/j.ydbio.2014.08.014. Epub 2014 Aug 23. Dev Biol. 2014. PMID: 25158168 Free PMC article.
-
Heterotypic control of basement membrane dynamics during branching morphogenesis.Dev Biol. 2015 May 1;401(1):103-9. doi: 10.1016/j.ydbio.2014.12.011. Epub 2014 Dec 16. Dev Biol. 2015. PMID: 25527075 Free PMC article. Review.
-
Cell adhesion: the molecular basis of tissue architecture and morphogenesis.Cell. 1996 Feb 9;84(3):345-57. doi: 10.1016/s0092-8674(00)81279-9. Cell. 1996. PMID: 8608588 Review.
Cited by
-
ARHGEF9 regulates melanoma morphogenesis in environments with diverse geometry and elasticity by promoting filopodial-driven adhesion.iScience. 2022 Aug 8;25(8):104795. doi: 10.1016/j.isci.2022.104795. eCollection 2022 Aug 19. iScience. 2022. PMID: 36039362 Free PMC article.
-
Laminin N-terminus α31 expression during development is lethal and causes widespread tissue-specific defects in a transgenic mouse model.FASEB J. 2022 Jul;36(7):e22318. doi: 10.1096/fj.202002588RRR. FASEB J. 2022. PMID: 35648586 Free PMC article.
-
Engineering clinically-relevant human fibroblastic cell-derived extracellular matrices.Methods Cell Biol. 2020;156:109-160. doi: 10.1016/bs.mcb.2019.11.014. Epub 2020 Jan 21. Methods Cell Biol. 2020. PMID: 32222216 Free PMC article.
-
The role of titanium surface micromorphology in MG-63 cell motility during osteogenesis.Sci Rep. 2022 Jun 15;12(1):9971. doi: 10.1038/s41598-022-13854-2. Sci Rep. 2022. PMID: 35705640 Free PMC article.
-
Cellular plasticity and fate determination in gastric carcinogenesis.iScience. 2024 Mar 8;27(4):109465. doi: 10.1016/j.isci.2024.109465. eCollection 2024 Apr 19. iScience. 2024. PMID: 38550991 Free PMC article. Review.
References
-
- Charras G, Sahai E. Physical influences of the extracellular environment on cell migration. Nat Rev Mol Cell Biol. 2014;15:813‐824. - PubMed
-
- Dzamba BJ, DeSimone DW. Extracellular matrix (ECM) and the sculpting of embryonic tissues. Curr Top Dev Biol. 2018;130:245‐274. - PubMed
-
- Gauthier NC, Roca‐Cusachs P. Mechanosensing at integrin‐mediated cell‐matrix adhesions: from molecular to integrated mechanisms. Curr Opin Cell Biol. 2018;50:20‐26. - PubMed
Publication types
MeSH terms
LinkOut - more resources
Full Text Sources