Silica nanoparticles induce autophagy dysfunction via lysosomal impairment and inhibition of autophagosome degradation in hepatocytes
- PMID: 28182147
- PMCID: PMC5279829
- DOI: 10.2147/IJN.S123596
Silica nanoparticles induce autophagy dysfunction via lysosomal impairment and inhibition of autophagosome degradation in hepatocytes
Abstract
Autophagy dysfunction is considered as a potential toxic mechanism of nanomaterials. Silica nanoparticles (SiNPs) can induce autophagy, but the specific mechanism involved remains unclear. Therefore, the aim of this study was to confirm the effects of SiNPs on autophagy dysfunction and explore the possible underlying mechanism. In this article, we reported that cell-internalized SiNPs exhibited dose- and time-dependent cytotoxicity in both L-02 and HepG2 cells. Multiple methods verified that SiNPs induced autophagy even at the noncytotoxic level and blocked the autophagic flux at the high-dose level. Notably, SiNPs impaired the lysosomal function through damaging lysosomal ultrastructures, increasing membrane permeability, and downregulating the expression of lysosomal proteases, cathepsin B, as evidenced by transmission electron microscopy, acridine orange staining, quantitative reverse transcription-polymerase chain reaction, and Western blot assays. Collectively, these data concluded that SiNPs inhibited autophagosome degradation via lysosomal impairment in hepatocytes, resulting in autophagy dysfunction. The current study not only discloses a potential mechanism of autophagy dysfunction induced by SiNPs but also provides novel evidence for the study of toxic effect and safety evaluation of SiNPs.
Keywords: autophagosome; autophagy dysfunction; hepatocytes; lysosome; silica nanoparticles.
Conflict of interest statement
The authors report no conflicts of interest in this work.
Figures
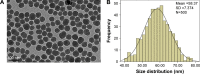
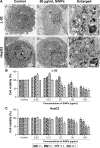
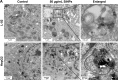
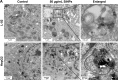
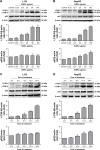
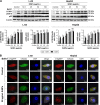
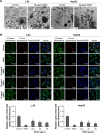
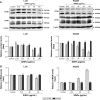
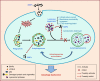
Similar articles
-
Silica nanoparticles induce autophagosome accumulation via activation of the EIF2AK3 and ATF6 UPR pathways in hepatocytes.Autophagy. 2018;14(7):1185-1200. doi: 10.1080/15548627.2018.1458174. Epub 2018 Jul 20. Autophagy. 2018. PMID: 29940794 Free PMC article.
-
Autophagic flux blockage in alveolar epithelial cells is essential in silica nanoparticle-induced pulmonary fibrosis.Cell Death Dis. 2019 Feb 12;10(2):127. doi: 10.1038/s41419-019-1340-8. Cell Death Dis. 2019. PMID: 30755584 Free PMC article.
-
Silica nanoparticles induce lung inflammation in mice via ROS/PARP/TRPM2 signaling-mediated lysosome impairment and autophagy dysfunction.Part Fibre Toxicol. 2020 Jun 8;17(1):23. doi: 10.1186/s12989-020-00353-3. Part Fibre Toxicol. 2020. PMID: 32513195 Free PMC article.
-
The toxicity of silica nanoparticles to the immune system.Nanomedicine (Lond). 2018 Aug 1;13(15):1939-1962. doi: 10.2217/nnm-2018-0076. Epub 2018 Aug 28. Nanomedicine (Lond). 2018. PMID: 30152253 Review.
-
Toxicology of silica nanoparticles: an update.Arch Toxicol. 2017 Sep;91(9):2967-3010. doi: 10.1007/s00204-017-1993-y. Epub 2017 Jun 1. Arch Toxicol. 2017. PMID: 28573455 Free PMC article. Review.
Cited by
-
Chemosensitivity enhanced by autophagy inhibition based on a polycationic nano-drug carrier.Nanoscale Adv. 2021 Jan 27;3(6):1656-1673. doi: 10.1039/d0na00990c. eCollection 2021 Mar 23. Nanoscale Adv. 2021. PMID: 36132550 Free PMC article.
-
Enhancing Therapeutic Efficacy in Cancer Treatment: Integrating Nanomedicine with Autophagy Inhibition Strategies.ACS Omega. 2024 Jun 18;9(26):27832-27852. doi: 10.1021/acsomega.4c02234. eCollection 2024 Jul 2. ACS Omega. 2024. PMID: 38973850 Free PMC article. Review.
-
Nano-hydroxyapatite accelerates vascular calcification via lysosome impairment and autophagy dysfunction in smooth muscle cells.Bioact Mater. 2021 Jun 14;8:478-493. doi: 10.1016/j.bioactmat.2021.06.004. eCollection 2022 Feb. Bioact Mater. 2021. PMID: 34541414 Free PMC article.
-
Unexpected Inhibitory Role of Silica Nanoparticles on Lung Cancer Development by Promoting M1 Polarization of Macrophages.Int J Nanomedicine. 2024 Nov 1;19:11087-11104. doi: 10.2147/IJN.S472796. eCollection 2024. Int J Nanomedicine. 2024. PMID: 39502640 Free PMC article.
-
Autophagy: a necessary evil in cancer and inflammation.3 Biotech. 2024 Mar;14(3):87. doi: 10.1007/s13205-023-03864-w. Epub 2024 Feb 20. 3 Biotech. 2024. PMID: 38390576 Free PMC article. Review.
References
-
- Wang Y, Zhao Q, Han N, et al. Mesoporous silica nanoparticles in drug delivery and biomedical applications. Nanomedicine. 2015;11(2):313–327. - PubMed
-
- Yang Y, Li J. Lipid, protein and poly(NIPAM) coated mesoporous silica nanoparticles for biomedical applications. Adv Colloid Interface Sci. 2014;207:155–163. - PubMed
-
- Mai WX, Meng H. Mesoporous silica nanoparticles: a multifunctional nano therapeutic system. Integr Biol (Camb) 2013;5(1):19–28. - PubMed
MeSH terms
Substances
LinkOut - more resources
Full Text Sources
Other Literature Sources