A substrate preference for the rough endoplasmic reticulum resident protein FKBP22 during collagen biosynthesis
- PMID: 24821723
- PMCID: PMC4140264
- DOI: 10.1074/jbc.M114.561944
A substrate preference for the rough endoplasmic reticulum resident protein FKBP22 during collagen biosynthesis
Abstract
The biosynthesis of collagens occurs in the rough endoplasmic reticulum and requires a large numbers of molecular chaperones, foldases, and post-translational modification enzymes. Collagens contain a large number of proline residues that are post-translationally modified to 3-hydroxyproline or 4-hydroxyproline, and the rate-limiting step in formation of the triple helix is the cis-trans isomerization of peptidyl-proline bonds. This step is catalyzed by peptidyl-prolyl cis-trans isomerases. There are seven peptidyl-prolyl cis-trans isomerases in the rER, and so far, two of these enzymes, cyclophilin B and FKBP65, have been shown to be involved in collagen biosynthesis. The absence of either cyclophilin B or FKBP65 leads to a recessive form of osteogenesis imperfecta. The absence of FKBP22 leads to a kyphoscoliotic type of Ehlers-Danlos syndrome (EDS), and this type of EDS is classified as EDS type VI, which can also be caused by a deficiency in lysyl-hydroxylase 1. However, the lack of FKBP22 shows a wider spectrum of clinical phenotypes than the absence of lysyl-hydroxylase 1 and additionally includes myopathy, hearing loss, and aortic rupture. Here we show that FKBP22 catalyzes the folding of type III collagen and interacts with type III collagen, type VI collagen, and type X collagen, but not with type I collagen, type II collagen, or type V collagen. These restrictive interactions might help explain the broader phenotype observed in patients that lack FKBP22.
Keywords: Biosynthesis; Collagen; Endoplasmic Reticulum (ER); FK506-binding Protein; Molecular Chaperone; Peptidyl-Prolyl Cis-Trans Isomerase; Protein Folding.
© 2014 by The American Society for Biochemistry and Molecular Biology, Inc.
Figures
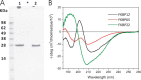
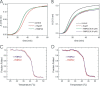
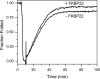
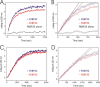
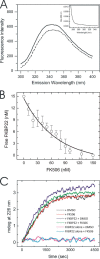
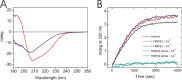
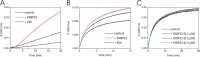
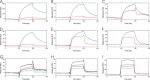
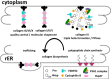
Similar articles
-
Ziploc-ing the structure 2.0: Endoplasmic reticulum-resident peptidyl prolyl isomerases show different activities toward hydroxyproline.J Biol Chem. 2017 Jun 2;292(22):9273-9282. doi: 10.1074/jbc.M116.772657. Epub 2017 Apr 6. J Biol Chem. 2017. PMID: 28385890 Free PMC article.
-
The rough endoplasmic reticulum-resident FK506-binding protein FKBP65 is a molecular chaperone that interacts with collagens.J Biol Chem. 2008 Nov 14;283(46):31584-90. doi: 10.1074/jbc.M802535200. Epub 2008 Sep 10. J Biol Chem. 2008. PMID: 18786928
-
Local Net Charge State of Collagen Triple Helix Is a Determinant of FKBP22 Binding to Collagen III.Int J Mol Sci. 2023 Oct 13;24(20):15156. doi: 10.3390/ijms242015156. Int J Mol Sci. 2023. PMID: 37894834 Free PMC article.
-
FK506-Binding protein 22 from a psychrophilic bacterium, a cold shock-inducible peptidyl prolyl isomerase with the ability to assist in protein folding.Int J Mol Sci. 2011;12(8):5261-84. doi: 10.3390/ijms12085261. Epub 2011 Aug 17. Int J Mol Sci. 2011. PMID: 21954357 Free PMC article. Review.
-
Ziploc-ing the structure: Triple helix formation is coordinated by rough endoplasmic reticulum resident PPIases.Biochim Biophys Acta. 2015 Oct;1850(10):1983-93. doi: 10.1016/j.bbagen.2014.12.024. Epub 2015 Jan 10. Biochim Biophys Acta. 2015. PMID: 25583561 Review.
Cited by
-
The emerging role of peptidyl-prolyl isomerase chaperones in tau oligomerization, amyloid processing, and Alzheimer's disease.J Neurochem. 2015 Apr;133(1):1-13. doi: 10.1111/jnc.13033. Epub 2015 Feb 24. J Neurochem. 2015. PMID: 25628064 Free PMC article. Review.
-
Ovol1/2 loss-induced epidermal defects elicit skin immune activation and alter global metabolism.EMBO Rep. 2023 Jul 5;24(7):e56214. doi: 10.15252/embr.202256214. Epub 2023 May 30. EMBO Rep. 2023. PMID: 37249012 Free PMC article.
-
Let Time Teach You: A Case Report of a Double Diagnosis of 17P Duplication and Ehlers-Danlos Syndrome.Genes (Basel). 2022 Nov 23;13(12):2197. doi: 10.3390/genes13122197. Genes (Basel). 2022. PMID: 36553464 Free PMC article.
-
Transcriptome analysis of skin fibroblasts with dominant negative COL3A1 mutations provides molecular insights into the etiopathology of vascular Ehlers-Danlos syndrome.PLoS One. 2018 Jan 18;13(1):e0191220. doi: 10.1371/journal.pone.0191220. eCollection 2018. PLoS One. 2018. PMID: 29346445 Free PMC article.
-
Cellular and Molecular Mechanisms in the Pathogenesis of Classical, Vascular, and Hypermobile Ehlers‒Danlos Syndromes.Genes (Basel). 2019 Aug 12;10(8):609. doi: 10.3390/genes10080609. Genes (Basel). 2019. PMID: 31409039 Free PMC article. Review.
References
-
- Bächinger H. P., Mizuno K., Vranka J., Boudko S. (2010) Collagen Formation and Structure. in Comprehensive Natural Products II: Chemistry and Biology (Mander L., Liu H.-W., eds) pp. 469–530, Elsevier, Oxford
-
- Bateman J. F., Boot-Handford R. P., Lamandé S. R. (2009) Genetic diseases of connective tissues: cellular and extracellular effects of ECM mutations. Nat. Rev. Genet. 10, 173–183 - PubMed
-
- Ishikawa Y., Bächinger H. P. (2013) A molecular ensemble in the rER for procollagen maturation. Biochim. Biophys. Acta 1833, 2479–2491 - PubMed
Publication types
MeSH terms
Substances
LinkOut - more resources
Full Text Sources
Other Literature Sources
Medical
Molecular Biology Databases
Research Materials