Molecular complexes that direct rhodopsin transport to primary cilia
- PMID: 24135424
- PMCID: PMC3883129
- DOI: 10.1016/j.preteyeres.2013.08.004
Molecular complexes that direct rhodopsin transport to primary cilia
Abstract
Rhodopsin is a key molecular constituent of photoreceptor cells, yet understanding of how it regulates photoreceptor membrane trafficking and biogenesis of light-sensing organelles, the rod outer segments (ROS) is only beginning to emerge. Recently identified sequence of well-orchestrated molecular interactions of rhodopsin with the functional networks of Arf and Rab GTPases at multiple stages of intracellular targeting fits well into the complex framework of the biogenesis and maintenance of primary cilia, of which the ROS is one example. This review will discuss the latest progress in dissecting the molecular complexes that coordinate rhodopsin incorporation into ciliary-targeted carriers with the recruitment and activation of membrane tethering complexes and regulators of fusion with the periciliary plasma membrane. In addition to revealing the fundamental principals of ciliary membrane renewal, recent advances also provide molecular insight into the ways by which disruptions of the exquisitely orchestrated interactions lead to cilia dysfunction and result in human retinal dystrophies and syndromic diseases that affect multiple organs, including the eyes.
Keywords: ADRP; Arfs; Autosomal Dominant Retinitis Pigmentosa; BBS; BBSome; Bardet-Biedl Syndrome; CTS; Ciliary Targeting Signal; Cilium; DHA; Docosahexaenoic Acid; GAP; GC1; GEF; GTPase Activating Protein; Guanine Nucleotide Exchange Factor; Guanylyl Cyclase 1; IFT; Intraflagellar Transport; JBTS; Joubert Syndrome; MKS; MT; MTOC; Meckel Syndrome; Microtubule Organizing Center; Microtubules; NPHP; Nephronophthisis; OCT; Optical Coherence Tomography; PLA; Proximity Ligation Assay; RIS; ROS; RPGR; RTC(s); Rabs; Retinitis Pigmentosa GTPase Regulator; Rhodopsin; Rhodopsin Transport Carrier(s); Rod Inner Segment(s); Rod Outer Segment(s); SNARE; Soluble N-ethylmaleimide-sensitive Factor Attachment Protein Receptor; TGN; Trafficking; Trans-Golgi Network; a conserved complex of BBS proteins.
Copyright © 2013 Elsevier Ltd. All rights reserved.
Figures
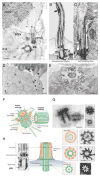
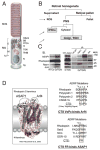
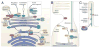
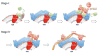
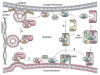
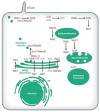
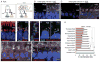
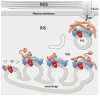
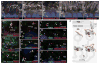
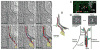
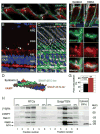
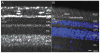
Similar articles
-
Molecular assemblies that control rhodopsin transport to the cilia.Vision Res. 2012 Dec 15;75:5-10. doi: 10.1016/j.visres.2012.07.015. Epub 2012 Aug 7. Vision Res. 2012. PMID: 22892112 Free PMC article. Review.
-
Syntaxin 3 and SNAP-25 pairing, regulated by omega-3 docosahexaenoic acid, controls the delivery of rhodopsin for the biogenesis of cilia-derived sensory organelles, the rod outer segments.J Cell Sci. 2009 Jun 15;122(Pt 12):2003-13. doi: 10.1242/jcs.039982. Epub 2009 May 19. J Cell Sci. 2009. PMID: 19454479 Free PMC article.
-
The Arf GAP ASAP1 provides a platform to regulate Arf4- and Rab11-Rab8-mediated ciliary receptor targeting.EMBO J. 2012 Oct 17;31(20):4057-71. doi: 10.1038/emboj.2012.253. Epub 2012 Sep 14. EMBO J. 2012. PMID: 22983554 Free PMC article.
-
Protein networks and complexes in photoreceptor cilia.Subcell Biochem. 2007;43:209-35. doi: 10.1007/978-1-4020-5943-8_10. Subcell Biochem. 2007. PMID: 17953396 Review.
-
The ins and outs of the Arf4-based ciliary membrane-targeting complex.Small GTPases. 2021 Jan;12(1):1-12. doi: 10.1080/21541248.2019.1616355. Epub 2019 May 17. Small GTPases. 2021. PMID: 31068062 Free PMC article. Review.
Cited by
-
Mitochondrial dysfunction in Spaceflight Associated Neuro-Ocular Syndrome (SANS): a molecular hypothesis in pathogenesis.Eye (Lond). 2024 Jun;38(8):1409-1411. doi: 10.1038/s41433-024-02951-3. Epub 2024 Feb 7. Eye (Lond). 2024. PMID: 38326485 Free PMC article. No abstract available.
-
Untangling ciliary access and enrichment of two rhodopsin-like receptors using quantitative fluorescence microscopy reveals cell-specific sorting pathways.Mol Biol Cell. 2017 Feb 15;28(4):554-566. doi: 10.1091/mbc.E16-07-0549. Epub 2016 Dec 14. Mol Biol Cell. 2017. PMID: 27974638 Free PMC article.
-
Structure and dynamics of photoreceptor sensory cilia.Pflugers Arch. 2021 Sep;473(9):1517-1537. doi: 10.1007/s00424-021-02564-9. Epub 2021 May 28. Pflugers Arch. 2021. PMID: 34050409 Free PMC article. Review.
-
Impact of reduced rhodopsin expression on the structure of rod outer segment disc membranes.Biochemistry. 2015 May 12;54(18):2885-94. doi: 10.1021/acs.biochem.5b00003. Epub 2015 Apr 27. Biochemistry. 2015. PMID: 25881629 Free PMC article.
-
Membrane association and remodeling by intraflagellar transport protein IFT172.Nat Commun. 2018 Nov 8;9(1):4684. doi: 10.1038/s41467-018-07037-9. Nat Commun. 2018. PMID: 30409972 Free PMC article.
References
-
- Alberts P, Galli T. The cell outgrowth secretory endosome (COSE): a specialized compartment involved in neuronal morphogenesis. Biol Cell. 2003;95:419–424. - PubMed
-
- Arts HH, Doherty D, van Beersum SE, Parisi MA, Letteboer SJ, Gorden NT, Peters TA, Marker T, Voesenek K, Kartono A, Ozyurek H, Farin FM, Kroes HY, Wolfrum U, Brunner HG, Cremers FP, Glass IA, Knoers NV, Roepman R. Mutations in the gene encoding the basal body protein RPGRIP1L, a nephrocystin-4 interactor, cause Joubert syndrome. Nat Genet. 2007;39:882–888. - PubMed
Publication types
MeSH terms
Substances
Grants and funding
LinkOut - more resources
Full Text Sources
Other Literature Sources
Research Materials
Miscellaneous