A novel dominant hyperekplexia mutation Y705C alters trafficking and biochemical properties of the presynaptic glycine transporter GlyT2
- PMID: 22753417
- PMCID: PMC3436537
- DOI: 10.1074/jbc.M111.319244
A novel dominant hyperekplexia mutation Y705C alters trafficking and biochemical properties of the presynaptic glycine transporter GlyT2
Abstract
Hyperekplexia or startle disease is characterized by an exaggerated startle response, evoked by tactile or auditory stimuli, producing hypertonia and apnea episodes. Although rare, this orphan disorder can have serious consequences, including sudden infant death. Dominant and recessive mutations in the human glycine receptor (GlyR) α1 gene (GLRA1) are the major cause of this disorder. However, recessive mutations in the presynaptic Na(+)/Cl(-)-dependent glycine transporter GlyT2 gene (SLC6A5) are rapidly emerging as a second major cause of startle disease. In this study, systematic DNA sequencing of SLC6A5 revealed a new dominant GlyT2 mutation: pY705C (c.2114A→G) in transmembrane domain 11, in eight individuals from Spain and the United Kingdom. Curiously, individuals harboring this mutation show significant variation in clinical presentation. In addition to classical hyperekplexia symptoms, some individuals had abnormal respiration, facial dysmorphism, delayed motor development, or intellectual disability. We functionally characterized this mutation using molecular modeling, electrophysiology, [(3)H]glycine transport, cell surface expression, and cysteine labeling assays. We found that the introduced cysteine interacts with the cysteine pair Cys-311-Cys-320 in the second external loop of GlyT2. This interaction impairs transporter maturation through the secretory pathway, reduces surface expression, and inhibits transport function. Additionally, Y705C presents altered H(+) and Zn(2+) dependence of glycine transport that may affect the function of glycinergic neurotransmission in vivo.
Figures
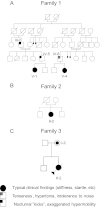
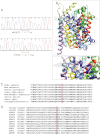
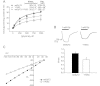
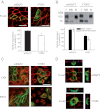
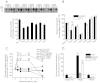
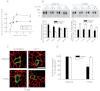
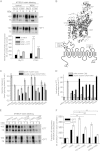
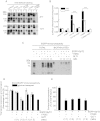
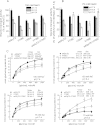
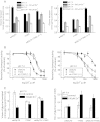
Similar articles
-
Molecular basis of the dominant negative effect of a glycine transporter 2 mutation associated with hyperekplexia.J Biol Chem. 2015 Jan 23;290(4):2150-65. doi: 10.1074/jbc.M114.587055. Epub 2014 Dec 5. J Biol Chem. 2015. PMID: 25480793 Free PMC article.
-
Mutations in the GlyT2 gene (SLC6A5) are a second major cause of startle disease.J Biol Chem. 2012 Aug 17;287(34):28975-85. doi: 10.1074/jbc.M112.372094. Epub 2012 Jun 14. J Biol Chem. 2012. PMID: 22700964 Free PMC article. Clinical Trial.
-
Mutations in the gene encoding GlyT2 (SLC6A5) define a presynaptic component of human startle disease.Nat Genet. 2006 Jul;38(7):801-6. doi: 10.1038/ng1814. Epub 2006 Jun 4. Nat Genet. 2006. PMID: 16751771 Free PMC article.
-
Hyperekplexia-associated mutations in the neuronal glycine transporter 2.Neurochem Int. 2019 Feb;123:95-100. doi: 10.1016/j.neuint.2018.05.014. Epub 2018 May 30. Neurochem Int. 2019. PMID: 29859229 Review.
-
Molecular mechanisms of glycine transporter GlyT2 mutations in startle disease.Biol Chem. 2012 Apr;393(4):283-9. doi: 10.1515/BC-2011-232. Biol Chem. 2012. PMID: 22114948 Review.
Cited by
-
Structural Determinants of the Neuronal Glycine Transporter 2 for the Selective Inhibitors ALX1393 and ORG25543.ACS Chem Neurosci. 2021 Jun 2;12(11):1860-1872. doi: 10.1021/acschemneuro.0c00602. Epub 2021 May 18. ACS Chem Neurosci. 2021. PMID: 34003005 Free PMC article.
-
A comparison of the transport kinetics of glycine transporter 1 and glycine transporter 2.J Gen Physiol. 2019 Aug 5;151(8):1035-1050. doi: 10.1085/jgp.201912318. Epub 2019 Jul 3. J Gen Physiol. 2019. PMID: 31270129 Free PMC article.
-
Molecular basis of the dominant negative effect of a glycine transporter 2 mutation associated with hyperekplexia.J Biol Chem. 2015 Jan 23;290(4):2150-65. doi: 10.1074/jbc.M114.587055. Epub 2014 Dec 5. J Biol Chem. 2015. PMID: 25480793 Free PMC article.
-
A cytosolic relay of heat shock proteins HSP70-1A and HSP90β monitors the folding trajectory of the serotonin transporter.J Biol Chem. 2014 Oct 17;289(42):28987-9000. doi: 10.1074/jbc.M114.595090. Epub 2014 Sep 8. J Biol Chem. 2014. PMID: 25202009 Free PMC article.
-
Distinct phenotypes in zebrafish models of human startle disease.Neurobiol Dis. 2013 Dec;60:139-51. doi: 10.1016/j.nbd.2013.09.002. Epub 2013 Sep 9. Neurobiol Dis. 2013. PMID: 24029548 Free PMC article.
References
-
- Aragón C., López-Corcuera B. (2003) Structure, function and regulation of glycine neurotransporters. Eur. J. Pharmacol. 479, 249–262 - PubMed
-
- Gomeza J., Hülsmann S., Ohno K., Eulenburg V., Szöke K., Richter D., Betz H. (2003) Inactivation of the glycine transporter 1 gene discloses vital role of glial glycine uptake in glycinergic inhibition. Neuron 40, 785–796 - PubMed
-
- Aragón C., López-Corcuera B. (2005) Glycine transporters. Crucial roles of pharmacological interest revealed by gene deletion. Trends Pharmacol. Sci. 26, 283–286 - PubMed
-
- Gomeza J., Ohno K., Hülsmann S., Armsen W., Eulenburg V., Richter D. W., Laube B., Betz H. (2003) Deletion of the mouse glycine transporter 2 results in a hyperekplexia phenotype and postnatal lethality. Neuron 40, 797–806 - PubMed
-
- Gomeza J., Ohno K., Betz H. (2003) Glycine transporter isoforms in the mammalian central nervous system. Structures, functions and therapeutic promises. Curr. Opin. Drug Discov. Devel. 6, 675–682 - PubMed
Publication types
MeSH terms
Substances
Grants and funding
LinkOut - more resources
Full Text Sources
Medical
Molecular Biology Databases