Eukaryote-like serine/threonine kinases and phosphatases in bacteria
- PMID: 21372323
- PMCID: PMC3063355
- DOI: 10.1128/MMBR.00042-10
Eukaryote-like serine/threonine kinases and phosphatases in bacteria
Abstract
Genomic studies have revealed the presence of Ser/Thr kinases and phosphatases in many bacterial species, although their physiological roles have largely been unclear. Here we review bacterial Ser/Thr kinases (eSTKs) that show homology in their catalytic domains to eukaryotic Ser/Thr kinases and their partner phosphatases (eSTPs) that are homologous to eukaryotic phosphatases. We first discuss insights into the enzymatic mechanism of eSTK activation derived from structural studies on both the ligand-binding and catalytic domains. We then turn our attention to the identified substrates of eSTKs and eSTPs for a number of species and to the implications of these findings for understanding their physiological roles in these organisms.
Figures
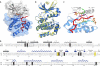
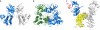
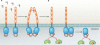
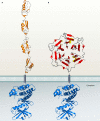
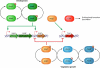
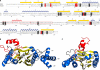
Similar articles
-
Ser/Thr phosphorylation as a regulatory mechanism in bacteria.Curr Opin Microbiol. 2015 Apr;24:47-52. doi: 10.1016/j.mib.2015.01.005. Epub 2015 Jan 24. Curr Opin Microbiol. 2015. PMID: 25625314 Free PMC article. Review.
-
Regulation of transcription by eukaryotic-like serine-threonine kinases and phosphatases in Gram-positive bacterial pathogens.Virulence. 2014;5(8):863-85. doi: 10.4161/21505594.2014.983404. Virulence. 2014. PMID: 25603430 Free PMC article. Review.
-
The Eukaryotic-Like Ser/Thr Kinase PrkC Regulates the Essential WalRK Two-Component System in Bacillus subtilis.PLoS Genet. 2015 Jun 23;11(6):e1005275. doi: 10.1371/journal.pgen.1005275. eCollection 2015 Jun. PLoS Genet. 2015. PMID: 26102633 Free PMC article.
-
Diversity in domain architectures of Ser/Thr kinases and their homologues in prokaryotes.BMC Genomics. 2005 Sep 19;6:129. doi: 10.1186/1471-2164-6-129. BMC Genomics. 2005. PMID: 16171520 Free PMC article.
-
Identification and Biochemical Characterization of a Novel Protein Phosphatase 2C-Like Ser/Thr Phosphatase in Escherichia coli.J Bacteriol. 2018 Aug 24;200(18):e00225-18. doi: 10.1128/JB.00225-18. Print 2018 Sep 15. J Bacteriol. 2018. PMID: 29967116 Free PMC article.
Cited by
-
Phosphorylation-dependent activation of the cell wall synthase PBP2a in Streptococcus pneumoniae by MacP.Proc Natl Acad Sci U S A. 2018 Mar 13;115(11):2812-2817. doi: 10.1073/pnas.1715218115. Epub 2018 Feb 27. Proc Natl Acad Sci U S A. 2018. PMID: 29487215 Free PMC article.
-
KdpD is a tandem serine histidine kinase that controls K+ pump KdpFABC transcriptionally and post-translationally.Nat Commun. 2024 Apr 15;15(1):3223. doi: 10.1038/s41467-024-47526-8. Nat Commun. 2024. PMID: 38622146 Free PMC article.
-
Mechanistic Understanding of Lanthipeptide Biosynthetic Enzymes.Chem Rev. 2017 Apr 26;117(8):5457-5520. doi: 10.1021/acs.chemrev.6b00591. Epub 2017 Jan 30. Chem Rev. 2017. PMID: 28135077 Free PMC article. Review.
-
Are antibacterial effects of non-antibiotic drugs random or purposeful because of a common evolutionary origin of bacterial and mammalian targets?Infection. 2021 Aug;49(4):569-589. doi: 10.1007/s15010-020-01547-9. Epub 2020 Dec 15. Infection. 2021. PMID: 33325009 Free PMC article. Review.
-
Impact of Serine/Threonine Protein Kinases on the Regulation of Sporulation in Bacillus subtilis.Front Microbiol. 2016 Apr 20;7:568. doi: 10.3389/fmicb.2016.00568. eCollection 2016. Front Microbiol. 2016. PMID: 27148245 Free PMC article. Review.
References
-
- Absalon, C., et al. 2009. CpgA, EF-Tu and the stressosome protein YezB are substrates of the Ser/Thr kinase/phosphatase couple, PrkC/PrpC, in Bacillus subtilis. Microbiology 155:932-943. - PubMed
-
- Adler, E., A. Donella-Deana, F. Arigoni, L. A. Pinna, and P. Stragler. 1997. Structural relationship between a bacterial developmental protein and eukaryotic PP2C protein phosphatases. Mol. Microbiol. 23:57-62. - PubMed
-
- Alexander, C., et al. 1995. Phosphorylation of elongation factor Tu prevents ternary complex formation. J. Biol. Chem. 270:14541-14547. - PubMed
Publication types
MeSH terms
Substances
Grants and funding
LinkOut - more resources
Full Text Sources
Other Literature Sources