Genetic and physiological evidence that oligodendrocyte gap junctions contribute to spatial buffering of potassium released during neuronal activity
- PMID: 17065440
- PMCID: PMC6674647
- DOI: 10.1523/JNEUROSCI.0304-06.2006
Genetic and physiological evidence that oligodendrocyte gap junctions contribute to spatial buffering of potassium released during neuronal activity
Abstract
Mice lacking the K+ channel Kir4.1 or both connexin32 (Cx32) and Cx47 exhibit myelin-associated vacuoles, raising the possibility that oligodendrocytes, and the connexins they express, contribute to recycling the K+ evolved during neuronal activity. To study this possibility, we first examined the effect of neuronal activity on the appearance of vacuoles in mice lacking both Cx32 and Cx47. The size and number of myelin vacuoles was dramatically increased when axonal activity was increased, by either a natural stimulus (eye opening) or pharmacological treatment. Conversely, myelin vacuoles were dramatically reduced when axonal activity was suppressed. Second, we used genetic complementation to test for a relationship between the function of Kir4.1 and oligodendrocyte connexins. In a Cx32-null background, haploinsufficiency of either Cx47 or Kir4.1 did not affect myelin, but double heterozygotes developed vacuoles, consistent with the idea that oligodendrocyte connexins and Kir4.1 function in a common pathway. Together, these results implicate oligodendrocytes and their connexins as having critical roles in the buffering of K+ released during neuronal activity.
Figures
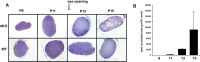
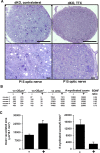
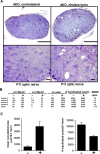
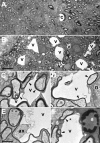
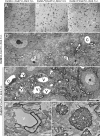
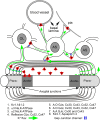
Similar articles
-
Connexin47, connexin29 and connexin32 co-expression in oligodendrocytes and Cx47 association with zonula occludens-1 (ZO-1) in mouse brain.Neuroscience. 2004;126(3):611-30. doi: 10.1016/j.neuroscience.2004.03.063. Neuroscience. 2004. PMID: 15183511 Free PMC article.
-
Ablation of Cx47 in transgenic mice leads to the loss of MUPP1, ZONAB and multiple connexins at oligodendrocyte-astrocyte gap junctions.Eur J Neurosci. 2008 Oct;28(8):1503-17. doi: 10.1111/j.1460-9568.2008.06431.x. Eur J Neurosci. 2008. PMID: 18973575 Free PMC article.
-
Connexin29 and connexin32 at oligodendrocyte and astrocyte gap junctions and in myelin of the mouse central nervous system.J Comp Neurol. 2003 Sep 22;464(3):356-70. doi: 10.1002/cne.10797. J Comp Neurol. 2003. PMID: 12900929 Free PMC article.
-
Severe Convulsions and Dysmyelination in Both Jimpy and Cx32/47 -/- Mice may Associate Astrocytic L-Channel Function with Myelination and Oligodendrocytic Connexins with Internodal Kv Channels.Neurochem Res. 2017 Jun;42(6):1747-1766. doi: 10.1007/s11064-017-2194-z. Epub 2017 Feb 18. Neurochem Res. 2017. PMID: 28214987 Review.
-
Inwardly Rectifying Potassium Channel Kir4.1 as a Novel Modulator of BDNF Expression in Astrocytes.Int J Mol Sci. 2018 Oct 24;19(11):3313. doi: 10.3390/ijms19113313. Int J Mol Sci. 2018. PMID: 30356026 Free PMC article. Review.
Cited by
-
Inherited and acquired disorders of myelin: The underlying myelin pathology.Exp Neurol. 2016 Sep;283(Pt B):452-75. doi: 10.1016/j.expneurol.2016.04.002. Epub 2016 Apr 9. Exp Neurol. 2016. PMID: 27068622 Free PMC article. Review.
-
Oligodendroglia and Myelin in Neurodegenerative Diseases: More Than Just Bystanders?Mol Neurobiol. 2016 Jul;53(5):3046-3062. doi: 10.1007/s12035-015-9205-3. Epub 2015 May 13. Mol Neurobiol. 2016. PMID: 25966971 Free PMC article. Review.
-
Leukoencephalopathy upon disruption of the chloride channel ClC-2.J Neurosci. 2007 Jun 13;27(24):6581-9. doi: 10.1523/JNEUROSCI.0338-07.2007. J Neurosci. 2007. PMID: 17567819 Free PMC article.
-
Synaptically silent sensory hair cells in zebrafish are recruited after damage.Nat Commun. 2018 Apr 11;9(1):1388. doi: 10.1038/s41467-018-03806-8. Nat Commun. 2018. PMID: 29643351 Free PMC article.
-
Gap junctions couple astrocytes and oligodendrocytes.J Mol Neurosci. 2008 May;35(1):101-16. doi: 10.1007/s12031-007-9027-5. J Mol Neurosci. 2008. PMID: 18236012 Free PMC article. Review.
References
-
- Amiry-Moghaddam M, Williamson A, Palomba M, Eid T, de Lanerolle NC, Nagelhus EA, Adams ME, Froehner SC, Agre P, Ottersen OP. Delayed K+ clearance associated with aquaporin-4 mislocalization: phenotypic defects in brains of alpha-syntrophin-null mice. Proc Natl Acad Sci USA. 2003;100:13615–13620. - PMC - PubMed
-
- Arroyo EJ, Scherer SS. On the molecular architecture of myelinated fibers. Histochem Cell Biol. 2000;113:1–18. - PubMed
Publication types
MeSH terms
Substances
Grants and funding
LinkOut - more resources
Full Text Sources
Medical
Molecular Biology Databases
Miscellaneous