The 2.2 A resolution structure of RpiB/AlsB from Escherichia coli illustrates a new approach to the ribose-5-phosphate isomerase reaction
- PMID: 14499611
- PMCID: PMC2792017
- DOI: 10.1016/j.jmb.2003.08.009
The 2.2 A resolution structure of RpiB/AlsB from Escherichia coli illustrates a new approach to the ribose-5-phosphate isomerase reaction
Abstract
Ribose-5-phosphate isomerases (EC 5.3.1.6) interconvert ribose 5-phosphate and ribulose 5-phosphate. This reaction permits the synthesis of ribose from other sugars, as well as the recycling of sugars from nucleotide breakdown. Two unrelated types of enzyme can catalyze the reaction. The most common, RpiA, is present in almost all organisms (including Escherichia coli), and is highly conserved. The second type, RpiB, is present in some bacterial and eukaryotic species and is well conserved. In E.coli, RpiB is sometimes referred to as AlsB, because it can take part in the metabolism of the rare sugar, allose, as well as the much more common ribose sugars. We report here the structure of RpiB/AlsB from E.coli, solved by multi-wavelength anomalous diffraction (MAD) phasing, and refined to 2.2A resolution. RpiB is the first structure to be solved from pfam02502 (the RpiB/LacAB family). It exhibits a Rossmann-type alphabetaalpha-sandwich fold that is common to many nucleotide-binding proteins, as well as other proteins with different functions. This structure is quite distinct from that of the previously solved RpiA; although both are, to some extent, based on the Rossmann fold, their tertiary and quaternary structures are very different. The four molecules in the RpiB asymmetric unit represent a dimer of dimers. Active-site residues were identified at the interface between the subunits, such that each active site has contributions from both subunits. Kinetic studies indicate that RpiB is nearly as efficient as RpiA, despite its completely different catalytic machinery. The sequence and structural results further suggest that the two homologous components of LacAB (galactose-6-phosphate isomerase) will compose a bi-functional enzyme; the second activity is unknown.
Figures
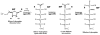
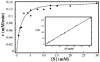
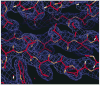
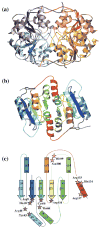
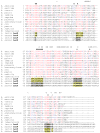
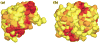
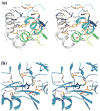
Similar articles
-
Mycobacterium tuberculosis ribose-5-phosphate isomerase has a known fold, but a novel active site.J Mol Biol. 2004 Jan 16;335(3):799-809. doi: 10.1016/j.jmb.2003.11.021. J Mol Biol. 2004. PMID: 14687575
-
Structure of Escherichia coli ribose-5-phosphate isomerase: a ubiquitous enzyme of the pentose phosphate pathway and the Calvin cycle.Structure. 2003 Jan;11(1):31-42. doi: 10.1016/s0969-2126(02)00933-4. Structure. 2003. PMID: 12517338 Free PMC article.
-
Structures of type B ribose 5-phosphate isomerase from Trypanosoma cruzi shed light on the determinants of sugar specificity in the structural family.FEBS J. 2011 Mar;278(5):793-808. doi: 10.1111/j.1742-4658.2010.07999.x. Epub 2011 Jan 25. FEBS J. 2011. PMID: 21205211
-
Engineering ribose-5-phosphate isomerase B from a central carbon metabolic enzyme to a promising sugar biocatalyst.Appl Microbiol Biotechnol. 2021 Jan;105(2):509-523. doi: 10.1007/s00253-020-11075-z. Epub 2021 Jan 4. Appl Microbiol Biotechnol. 2021. PMID: 33394147 Review.
-
Genetics of pentose-phosphate pathway enzymes of Escherichia coli K-12.Arch Microbiol. 1995 Nov;164(5):324-30. doi: 10.1007/BF02529978. Arch Microbiol. 1995. PMID: 8572885 Review.
Cited by
-
Chapter 4. Predicting and characterizing protein functions through matching geometric and evolutionary patterns of binding surfaces.Adv Protein Chem Struct Biol. 2008;75:107-41. doi: 10.1016/S0065-3233(07)75004-0. Epub 2009 Feb 26. Adv Protein Chem Struct Biol. 2008. PMID: 20731991 Free PMC article. Review.
-
A catalog of the diversity and ubiquity of bacterial microcompartments.Nat Commun. 2021 Jun 21;12(1):3809. doi: 10.1038/s41467-021-24126-4. Nat Commun. 2021. PMID: 34155212 Free PMC article.
-
The CorA Mg2+ transporter is a homotetramer.J Bacteriol. 2004 Jul;186(14):4605-12. doi: 10.1128/JB.186.14.4605-4612.2004. J Bacteriol. 2004. PMID: 15231793 Free PMC article.
-
New surface contacts formed upon reductive lysine methylation: improving the probability of protein crystallization.Protein Sci. 2010 Jul;19(7):1395-404. doi: 10.1002/pro.420. Protein Sci. 2010. PMID: 20506323 Free PMC article.
-
Ribose 5-phosphate isomerase type B from Trypanosoma cruzi: kinetic properties and site-directed mutagenesis reveal information about the reaction mechanism.Biochem J. 2007 Jan 1;401(1):279-85. doi: 10.1042/BJ20061049. Biochem J. 2007. PMID: 16981853 Free PMC article.
References
-
- David J, Wiesmeyer H. Regulation of ribose metabolism in Escherichia coli. II. Evidence for two ribose-5-phosphate isomerase activities. Biochim Biophys Acta. 1970;208:56–67. - PubMed
-
- Essenberg MK, Cooper RA. Two ribose-5-phosphate isomerases from Escherichia coli K12: partial characterisation of the enzymes and consideration of their possible physiological roles. Eur J Biochem. 1975;55:323–332. - PubMed
-
- Skinner AJ, Cooper RA. The regulation of ribose-5-phosphate isomerization in Escherichia coli K12. FEBS Letters. 1971;12:293–296. - PubMed
Publication types
MeSH terms
Substances
Associated data
- Actions
Grants and funding
LinkOut - more resources
Full Text Sources
Other Literature Sources
Molecular Biology Databases