The Power of Field-Flow Fractionation in Characterization of Nanoparticles in Drug Delivery
- PMID: 37241911
- PMCID: PMC10224342
- DOI: 10.3390/molecules28104169
The Power of Field-Flow Fractionation in Characterization of Nanoparticles in Drug Delivery
Abstract
Asymmetric-flow field-flow fractionation (AF4) is a gentle, flexible, and powerful separation technique that is widely utilized for fractionating nanometer-sized analytes, which extend to many emerging nanocarriers for drug delivery, including lipid-, virus-, and polymer-based nanoparticles. To ascertain quality attributes and suitability of these nanostructures as drug delivery systems, including particle size distributions, shape, morphology, composition, and stability, it is imperative that comprehensive analytical tools be used to characterize the native properties of these nanoparticles. The capacity for AF4 to be readily coupled to multiple online detectors (MD-AF4) or non-destructively fractionated and analyzed offline make this technique broadly compatible with a multitude of characterization strategies, which can provide insight on size, mass, shape, dispersity, and many other critical quality attributes. This review will critically investigate MD-AF4 reports for characterizing nanoparticles in drug delivery, especially those reported in the last 10-15 years that characterize multiple attributes simultaneously downstream from fractionation.
Keywords: asymmetrical flow field-flow fractionation; light scattering detection; multi-attribute characterization; nanoparticle drug delivery systems.
Conflict of interest statement
Author Juan Bian and Jessica Lin were employed by the company Genentech Inc. Author Nemal Gobalasingham and Anatolii Purchel were employed by the company Wyatt Technology. The authors declare no conflict of interest.
Figures


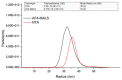
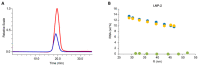
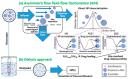
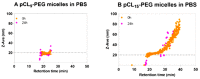
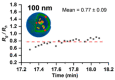
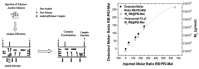
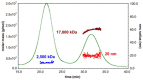
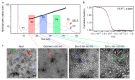
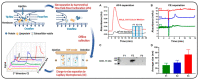
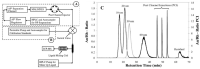
Similar articles
-
Development of an advanced separation and characterization platform for mRNA and lipid nanoparticles using multi-detector asymmetrical flow field-flow fractionation.Anal Bioanal Chem. 2024 Oct;416(24):5281-5293. doi: 10.1007/s00216-024-05455-x. Epub 2024 Aug 5. Anal Bioanal Chem. 2024. PMID: 39102094
-
Improved multidetector asymmetrical-flow field-flow fractionation method for particle sizing and concentration measurements of lipid-based nanocarriers for RNA delivery.Eur J Pharm Biopharm. 2021 Jun;163:252-265. doi: 10.1016/j.ejpb.2021.03.004. Epub 2021 Mar 18. Eur J Pharm Biopharm. 2021. PMID: 33745980
-
Measuring Particle Size Distribution by Asymmetric Flow Field Flow Fractionation: A Powerful Method for the Preclinical Characterization of Lipid-Based Nanoparticles.Mol Pharm. 2019 Feb 4;16(2):756-767. doi: 10.1021/acs.molpharmaceut.8b01033. Epub 2019 Jan 16. Mol Pharm. 2019. PMID: 30604620 Free PMC article.
-
Asymmetric flow field-flow fractionation as a multifunctional technique for the characterization of polymeric nanocarriers.Drug Deliv Transl Res. 2021 Apr;11(2):373-395. doi: 10.1007/s13346-021-00918-5. Epub 2021 Jan 31. Drug Deliv Transl Res. 2021. PMID: 33521866 Free PMC article. Review.
-
Asymmetric-flow field-flow fractionation for measuring particle size, drug loading and (in)stability of nanopharmaceuticals. The joint view of European Union Nanomedicine Characterization Laboratory and National Cancer Institute - Nanotechnology Characterization Laboratory.J Chromatogr A. 2021 Jan 4;1635:461767. doi: 10.1016/j.chroma.2020.461767. Epub 2020 Nov 27. J Chromatogr A. 2021. PMID: 33310281 Review.
Cited by
-
An in-depth physicochemical investigation of drug-loaded core-shell UiO66 nanoMOFs.RSC Adv. 2024 Jan 5;14(3):1676-1685. doi: 10.1039/d3ra07098k. eCollection 2024 Jan 3. RSC Adv. 2024. PMID: 38187455 Free PMC article.
-
Impact of Protein Nanoparticle Shape on the Immunogenicity of Antimicrobial Glycoconjugate Vaccines.Int J Mol Sci. 2024 Mar 27;25(7):3736. doi: 10.3390/ijms25073736. Int J Mol Sci. 2024. PMID: 38612547 Free PMC article.
-
Nanoscale Extracellular Vesicle-Enabled Liquid Biopsy: Advances and Challenges for Lung Cancer Detection.Micromachines (Basel). 2024 Sep 24;15(10):1181. doi: 10.3390/mi15101181. Micromachines (Basel). 2024. PMID: 39459055 Free PMC article. Review.
-
Development of an advanced separation and characterization platform for mRNA and lipid nanoparticles using multi-detector asymmetrical flow field-flow fractionation.Anal Bioanal Chem. 2024 Oct;416(24):5281-5293. doi: 10.1007/s00216-024-05455-x. Epub 2024 Aug 5. Anal Bioanal Chem. 2024. PMID: 39102094
References
-
- Patra J.K., Das G., Fraceto L.F., Campos E.V.R., Rodriguez-Torres M.D.P., Acosta-Torres L.S., Diaz-Torres L.A., Grillo R., Swamy M.K., Sharma S., et al. Nano based drug delivery systems: Recent developments and future prospects. J. Nanobiotechnol. 2018;16:71. doi: 10.1186/s12951-018-0392-8. - DOI - PMC - PubMed
Publication types
MeSH terms
Substances
Grants and funding
LinkOut - more resources
Full Text Sources