Lessons learned from immunological characterization of nanomaterials at the Nanotechnology Characterization Laboratory
- PMID: 36304452
- PMCID: PMC9592561
- DOI: 10.3389/fimmu.2022.984252
Lessons learned from immunological characterization of nanomaterials at the Nanotechnology Characterization Laboratory
Abstract
Nanotechnology carriers have become common in pharmaceutical products because of their benefits to drug delivery, including reduced toxicities and improved efficacy of active pharmaceutical ingredients due to targeted delivery, prolonged circulation time, and controlled payload release. While available examples of reduced drug toxicity through formulation using a nanocarrier are encouraging, current data also demonstrate that nanoparticles may change a drug's biodistribution and alter its toxicity profile. Moreover, individual components of nanoparticles and excipients commonly used in formulations are often not immunologically inert and contribute to the overall immune responses to nanotechnology-formulated products. Said immune responses may be beneficial or adverse depending on the indication, dose, dose regimen, and route of administration. Therefore, comprehensive toxicology studies are of paramount importance even when previously known drugs, components, and excipients are used in nanoformulations. Recent data also suggest that, despite decades of research directed at hiding nanocarriers from the immune recognition, the immune system's inherent property of clearing particulate materials can be leveraged to improve the therapeutic efficacy of drugs formulated using nanoparticles. Herein, I review current knowledge about nanoparticles' interaction with the immune system and how these interactions contribute to nanotechnology-formulated drug products' safety and efficacy through the lens of over a decade of nanoparticle characterization at the Nanotechnology Characterization Laboratory.
Keywords: characterization; immunotoxicity; methods; nanoparticles; nucleic acids; trends.
Copyright © 2022 Dobrovolskaia.
Conflict of interest statement
The author declares that the research was conducted in the absence of any commercial or financial relationships that could be construed as a potential conflict of interest.
Figures
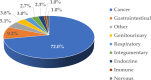
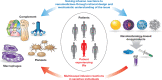
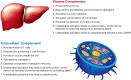
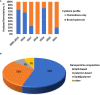
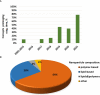
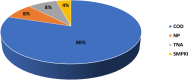
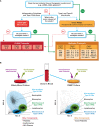
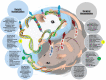
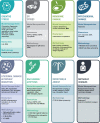
Similar articles
-
Strategy for selecting nanotechnology carriers to overcome immunological and hematological toxicities challenging clinical translation of nucleic acid-based therapeutics.Expert Opin Drug Deliv. 2015 Jul;12(7):1163-75. doi: 10.1517/17425247.2015.1042857. Epub 2015 May 20. Expert Opin Drug Deliv. 2015. PMID: 25994601 Review.
-
Current understanding of interactions between nanoparticles and the immune system.Toxicol Appl Pharmacol. 2016 May 15;299:78-89. doi: 10.1016/j.taap.2015.12.022. Epub 2015 Dec 29. Toxicol Appl Pharmacol. 2016. PMID: 26739622 Free PMC article. Review.
-
Nanosize drug delivery system.Curr Pharm Biotechnol. 2013;14(15):1221. doi: 10.2174/138920101415140804121008. Curr Pharm Biotechnol. 2013. PMID: 25106648
-
Recent update of toxicity aspects of nanoparticulate systems for drug delivery.Eur J Pharm Biopharm. 2021 Apr;161:100-119. doi: 10.1016/j.ejpb.2021.02.010. Epub 2021 Feb 25. Eur J Pharm Biopharm. 2021. PMID: 33639254 Review.
-
Nanotechnology in drug and gene delivery.Naunyn Schmiedebergs Arch Pharmacol. 2022 Jul;395(7):769-787. doi: 10.1007/s00210-022-02245-z. Epub 2022 May 4. Naunyn Schmiedebergs Arch Pharmacol. 2022. PMID: 35505234 Free PMC article. Review.
Cited by
-
Limitations of Limulus amebocyte lysate test for endotoxin control in raw materials for liposomal nanoformulations.Nanomedicine (Lond). 2024;19(27):2289-2300. doi: 10.1080/17435889.2024.2395243. Epub 2024 Oct 9. Nanomedicine (Lond). 2024. PMID: 39381981
-
In vitro assessment of nanomedicines' propensity to cause palmar-plantar erythrodysesthesia: A Doxil vs. doxorubicin case study.Nanomedicine. 2024 Nov;62:102780. doi: 10.1016/j.nano.2024.102780. Epub 2024 Aug 22. Nanomedicine. 2024. PMID: 39181221
-
Detection of Intracellular Complement Activation by Nanoparticles in Human T Lymphocytes.Methods Mol Biol. 2024;2789:109-120. doi: 10.1007/978-1-0716-3786-9_11. Methods Mol Biol. 2024. PMID: 38506996
-
Immunological properties of silica nanoparticles: a structure-activity relationship study.Nanotoxicology. 2024 Sep;18(6):542-564. doi: 10.1080/17435390.2024.2401448. Epub 2024 Sep 16. Nanotoxicology. 2024. PMID: 39282894
-
Rational Engineering of Islet Tolerance via Biomaterial-Mediated Immune Modulation.J Immunol. 2024 Jan 15;212(2):216-224. doi: 10.4049/jimmunol.2300527. J Immunol. 2024. PMID: 38166244 Free PMC article. Review.
References
Publication types
MeSH terms
Substances
Grants and funding
LinkOut - more resources
Full Text Sources