Fast-decaying plant litter enhances soil carbon in temperate forests but not through microbial physiological traits
- PMID: 35264580
- PMCID: PMC8907208
- DOI: 10.1038/s41467-022-28715-9
Fast-decaying plant litter enhances soil carbon in temperate forests but not through microbial physiological traits
Abstract
Conceptual and empirical advances in soil biogeochemistry have challenged long-held assumptions about the role of soil micro-organisms in soil organic carbon (SOC) dynamics; yet, rigorous tests of emerging concepts remain sparse. Recent hypotheses suggest that microbial necromass production links plant inputs to SOC accumulation, with high-quality (i.e., rapidly decomposing) plant litter promoting microbial carbon use efficiency, growth, and turnover leading to more mineral stabilization of necromass. We test this hypothesis experimentally and with observations across six eastern US forests, using stable isotopes to measure microbial traits and SOC dynamics. Here we show, in both studies, that microbial growth, efficiency, and turnover are negatively (not positively) related to mineral-associated SOC. In the experiment, stimulation of microbial growth by high-quality litter enhances SOC decomposition, offsetting the positive effect of litter quality on SOC stabilization. We suggest that microbial necromass production is not the primary driver of SOC persistence in temperate forests. Factors such as microbial necromass origin, alternative SOC formation pathways, priming effects, and soil abiotic properties can strongly decouple microbial growth, efficiency, and turnover from mineral-associated SOC.
© 2022. This is a U.S. Government work and not under copyright protection in the US; foreign copyright protection may apply.
Conflict of interest statement
The authors declare no competing interests.
Figures
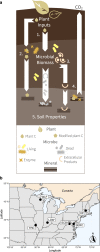
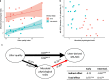
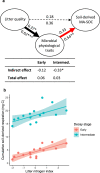
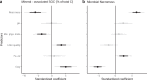
Similar articles
-
Evidence for the primacy of living root inputs, not root or shoot litter, in forming soil organic carbon.New Phytol. 2019 Jan;221(1):233-246. doi: 10.1111/nph.15361. Epub 2018 Aug 1. New Phytol. 2019. PMID: 30067293
-
Microbial and mineral interactions decouple litter quality from soil organic matter formation.Nat Commun. 2024 Nov 20;15(1):10063. doi: 10.1038/s41467-024-54446-0. Nat Commun. 2024. PMID: 39567513 Free PMC article.
-
Phosphorus addition decreases plant lignin but increases microbial necromass contribution to soil organic carbon in a subalpine forest.Glob Chang Biol. 2022 Jul;28(13):4194-4210. doi: 10.1111/gcb.16205. Epub 2022 May 2. Glob Chang Biol. 2022. PMID: 35445477
-
Research advance in the effects of litter input on forest soil organic carbon transformation and stability.Ying Yong Sheng Tai Xue Bao. 2024 Sep 18;35(9):2352-2361. doi: 10.13287/j.1001-9332.202409.033. Ying Yong Sheng Tai Xue Bao. 2024. PMID: 39435798 Review. English.
-
Clarifying the evidence for microbial- and plant-derived soil organic matter, and the path toward a more quantitative understanding.Glob Chang Biol. 2022 Dec;28(24):7167-7185. doi: 10.1111/gcb.16413. Epub 2022 Sep 15. Glob Chang Biol. 2022. PMID: 36043234 Review.
Cited by
-
Unveiling the deterministic dynamics of microbial meta-metabolism: a multi-omics investigation of anaerobic biodegradation.Microbiome. 2024 Sep 7;12(1):166. doi: 10.1186/s40168-024-01890-1. Microbiome. 2024. PMID: 39244624 Free PMC article.
-
Litter decomposition and nutrient release are faster under secondary forests than under Chinese fir plantations with forest development.Sci Rep. 2023 Oct 5;13(1):16805. doi: 10.1038/s41598-023-44042-5. Sci Rep. 2023. PMID: 37798470 Free PMC article.
-
Mineral states and sequestration processes involving soil biogenic components in various soils and desert sands of Inner Mongolia.Sci Rep. 2024 Nov 18;14(1):28530. doi: 10.1038/s41598-024-80004-1. Sci Rep. 2024. PMID: 39557936 Free PMC article.
-
The Impact and Determinants of Mountainous Topographical Factors on Soil Microbial Community Characteristics.Microorganisms. 2023 Nov 28;11(12):2878. doi: 10.3390/microorganisms11122878. Microorganisms. 2023. PMID: 38138022 Free PMC article.
-
Plant diversity drives soil carbon sequestration: evidence from 150 years of vegetation restoration in the temperate zone.Front Plant Sci. 2023 Jun 6;14:1191704. doi: 10.3389/fpls.2023.1191704. eCollection 2023. Front Plant Sci. 2023. PMID: 37346142 Free PMC article.
References
-
- Cotrufo MF, et al. Formation of soil organic matter via biochemical and physical pathways of litter mass loss. Nat. Geosci. 2015;8:776–779.
-
- Jilling A, et al. Minerals in the rhizosphere: overlooked mediators of soil nitrogen availability to plants and microbes. Biogeochemistry. 2018;139:103–122.
-
- Lal R. Soil Carbon Sequestration Impacts on Global Climate Change and Food Security. Science. 2004;304:1623–1627. - PubMed
-
- Lavallee JM, Soong JL, Cotrufo MF. Conceptualizing soil organic matter into particulate and mineral-associated forms to address global change in the 21st century. Glob. Change Biol. 2020;26:261–273. - PubMed
-
- Grandy AS, Neff JC. Molecular C dynamics downstream: the biochemical decomposition sequence and its impact on soil organic matter structure and function. Sci. Total Environ. 2008;404:297–307. - PubMed
Publication types
MeSH terms
Substances
LinkOut - more resources
Full Text Sources