Polymicrobial Interactions in the Cystic Fibrosis Airway Microbiome Impact the Antimicrobial Susceptibility of Pseudomonas aeruginosa
- PMID: 34356747
- PMCID: PMC8300716
- DOI: 10.3390/antibiotics10070827
Polymicrobial Interactions in the Cystic Fibrosis Airway Microbiome Impact the Antimicrobial Susceptibility of Pseudomonas aeruginosa
Abstract
Pseudomonas aeruginosa is one of the most dominant pathogens in cystic fibrosis (CF) airway disease and contributes to significant inflammation, airway damage, and poorer disease outcomes. The CF airway is now known to be host to a complex community of microorganisms, and polymicrobial interactions have been shown to play an important role in shaping P. aeruginosa pathogenicity and resistance. P. aeruginosa can cause chronic infections that once established are almost impossible to eradicate with antibiotics. CF patients that develop chronic P. aeruginosa infection have poorer lung function, higher morbidity, and a reduced life expectancy. P. aeruginosa adapts to the CF airway and quickly develops resistance to several antibiotics. A perplexing phenomenon is the disparity between in vitro antimicrobial sensitivity testing and clinical response. Considering the CF airway is host to a diverse community of microorganisms or 'microbiome' and that these microorganisms are known to interact, the antimicrobial resistance and progression of P. aeruginosa infection is likely influenced by these microbial relationships. This review combines the literature to date on interactions between P. aeruginosa and other airway microorganisms and the influence of these interactions on P. aeruginosa tolerance to antimicrobials.
Keywords: Pseudomonas aeruginosa; antibiotic resistance; cystic fibrosis; microbiome; polymicrobial interactions.
Conflict of interest statement
The authors declare no conflict of interest.
Figures
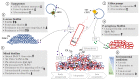
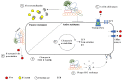
Similar articles
-
Pseudomonas aeruginosa Alters Staphylococcus aureus Sensitivity to Vancomycin in a Biofilm Model of Cystic Fibrosis Infection.mBio. 2017 Jul 18;8(4):e00873-17. doi: 10.1128/mBio.00873-17. mBio. 2017. PMID: 28720732 Free PMC article.
-
Disruption of Cross-Feeding Inhibits Pathogen Growth in the Sputa of Patients with Cystic Fibrosis.mSphere. 2020 Apr 29;5(2):e00343-20. doi: 10.1128/mSphere.00343-20. mSphere. 2020. PMID: 32350096 Free PMC article.
-
Tobramycin-Treated Pseudomonas aeruginosa PA14 Enhances Streptococcus constellatus 7155 Biofilm Formation in a Cystic Fibrosis Model System.J Bacteriol. 2015 Oct 19;198(2):237-47. doi: 10.1128/JB.00705-15. Print 2016 Jan 15. J Bacteriol. 2015. PMID: 26483523 Free PMC article.
-
Ecological Succession of Polymicrobial Communities in the Cystic Fibrosis Airways.mSystems. 2020 Dec 1;5(6):e00809-20. doi: 10.1128/mSystems.00809-20. mSystems. 2020. PMID: 33262240 Free PMC article. Review.
-
Combination antimicrobial susceptibility testing for acute exacerbations in chronic infection of Pseudomonas aeruginosa in cystic fibrosis.Cochrane Database Syst Rev. 2015 Nov 2;(11):CD006961. doi: 10.1002/14651858.CD006961.pub3. Cochrane Database Syst Rev. 2015. Update in: Cochrane Database Syst Rev. 2017 Jun 19;6:CD006961. doi: 10.1002/14651858.CD006961.pub4. PMID: 26522473 Updated. Review.
Cited by
-
Genetic determinants of antimicrobial resistance in polymyxin B resistant Pseudomonas aeruginosa isolated from airways of patients with cystic fibrosis.Braz J Microbiol. 2024 Jun;55(2):1415-1425. doi: 10.1007/s42770-024-01311-3. Epub 2024 Apr 15. Braz J Microbiol. 2024. PMID: 38619733
-
SARS-CoV-2 Infection in Patients with Cystic Fibrosis: What We Know So Far.Life (Basel). 2022 Dec 13;12(12):2087. doi: 10.3390/life12122087. Life (Basel). 2022. PMID: 36556452 Free PMC article. Review.
-
CFTR Modulator Therapies: Potential Impact on Airway Infections in Cystic Fibrosis.Cells. 2022 Apr 6;11(7):1243. doi: 10.3390/cells11071243. Cells. 2022. PMID: 35406809 Free PMC article. Review.
-
CFTR dysfunction leads to defective bacterial eradication on cystic fibrosis airways.Front Physiol. 2024 Apr 18;15:1385661. doi: 10.3389/fphys.2024.1385661. eCollection 2024. Front Physiol. 2024. PMID: 38699141 Free PMC article. Review.
-
Comparative analysis of antibiotic susceptibility patterns and clinical features of mucoid and non-mucoid Pseudomonas aeruginosa infections: a retrospective study.Front Public Health. 2024 Feb 8;12:1333477. doi: 10.3389/fpubh.2024.1333477. eCollection 2024. Front Public Health. 2024. PMID: 38389944 Free PMC article.
References
-
- CFF . Cystic Fibrosis Foundation 2018 Report. CFF; Bethesda, MD, USA: 2018.
-
- Elborn J.S., Bell S.C., Madge S.L., Burgel P.R., Castellani C., Conway S., De Rijcke K., Dembski B., Drevinek P., Heijerman H.G., et al. Report of the European Respiratory Society/European Cystic Fibrosis Society task force on the care of adults with cystic fibrosis. Eur. Respir. J. 2019;47:420–428. doi: 10.1183/13993003.00592-2015. - DOI - PubMed
Publication types
LinkOut - more resources
Full Text Sources