Antibody-Drug Conjugates: The Last Decade
- PMID: 32937862
- PMCID: PMC7558467
- DOI: 10.3390/ph13090245
Antibody-Drug Conjugates: The Last Decade
Abstract
An armed antibody (antibody-drug conjugate or ADC) is a vectorized chemotherapy, which results from the grafting of a cytotoxic agent onto a monoclonal antibody via a judiciously constructed spacer arm. ADCs have made considerable progress in 10 years. While in 2009 only gemtuzumab ozogamicin (Mylotarg®) was used clinically, in 2020, 9 Food and Drug Administration (FDA)-approved ADCs are available, and more than 80 others are in active clinical studies. This review will focus on FDA-approved and late-stage ADCs, their limitations including their toxicity and associated resistance mechanisms, as well as new emerging strategies to address these issues and attempt to widen their therapeutic window. Finally, we will discuss their combination with conventional chemotherapy or checkpoint inhibitors, and their design for applications beyond oncology, to make ADCs the magic bullet that Paul Ehrlich dreamed of.
Keywords: ADC; antibody–drug conjugate; bioconjugation; cancer; combination therapies; linker; payload; resistance.
Conflict of interest statement
Alain Beck is an employee of Pierre Fabre, Charles Dumontet has received research funding from Roche and is a founder of Mablinks. All authors have no relevant affiliations or financial involvement with any organization or entity with a financial interest in or financial conflict with the subject matter or materials discussed in the manuscript. This includes employment, consultancies, honoraria, stock ownership or options, expert testimony, grants or patents received or pending, or royalties.
Figures
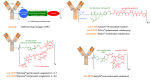
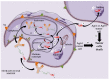
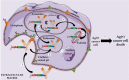
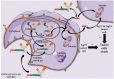
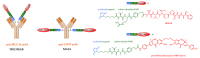
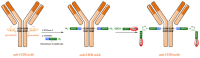
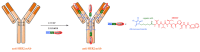
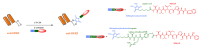
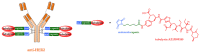
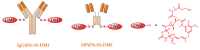
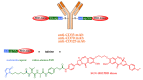
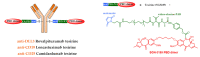
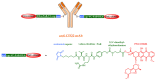
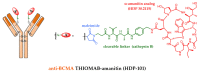
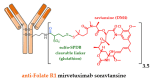
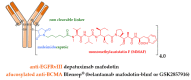
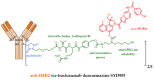
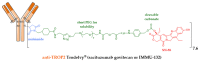
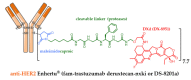
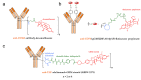
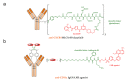
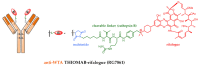
Similar articles
-
[Antibody-drug conjugates in oncology. New strategies in development].Med Sci (Paris). 2019 Dec;35(12):1043-1053. doi: 10.1051/medsci/2019228. Epub 2020 Jan 6. Med Sci (Paris). 2019. PMID: 31903916 Review. French.
-
[Antibody-drug conjugates in oncology. Recent success of an ancient concept].Med Sci (Paris). 2019 Dec;35(12):1034-1042. doi: 10.1051/medsci/2019227. Epub 2020 Jan 6. Med Sci (Paris). 2019. PMID: 31903915 Review. French.
-
Combining Biology and Chemistry for a New Take on Chemotherapy: Antibody-Drug Conjugates in Hematologic Malignancies.Curr Hematol Malig Rep. 2018 Dec;13(6):555-569. doi: 10.1007/s11899-018-0485-3. Curr Hematol Malig Rep. 2018. PMID: 30362019 Review.
-
Development, efficacy and side effects of antibody‑drug conjugates for cancer therapy (Review).Mol Clin Oncol. 2023 May 4;18(6):47. doi: 10.3892/mco.2023.2643. eCollection 2023 Jun. Mol Clin Oncol. 2023. PMID: 37206431 Free PMC article. Review.
-
Antibody-drug conjugates: Design and development for therapy and imaging in and beyond cancer, LabEx MAbImprove industrial workshop, July 27-28, 2017, Tours, France.MAbs. 2018 Feb/Mar;10(2):210-221. doi: 10.1080/19420862.2017.1412130. Epub 2018 Jan 9. MAbs. 2018. PMID: 29239690 Free PMC article.
Cited by
-
Extensive Biotransformation Profiling of AZD8205, an Anti-B7-H4 Antibody-Drug Conjugate, Elucidates Pathways Underlying Its Stability In Vivo.Anal Chem. 2024 Oct 22;96(42):16525-16533. doi: 10.1021/acs.analchem.4c02309. Epub 2024 Oct 11. Anal Chem. 2024. PMID: 39392424 Free PMC article.
-
Preclinical evaluation of a novel antibody-drug conjugate targeting DR5 for lymphoblastic leukemia therapy.Mol Ther Oncolytics. 2021 Apr 29;21:329-339. doi: 10.1016/j.omto.2021.04.013. eCollection 2021 Jun 25. Mol Ther Oncolytics. 2021. PMID: 34141870 Free PMC article.
-
Tumor-specific intracellular delivery: peptide-guided transport of a catalytic toxin.Commun Biol. 2023 Jan 17;6(1):60. doi: 10.1038/s42003-022-04385-7. Commun Biol. 2023. PMID: 36650239 Free PMC article.
-
Targeting the Gastrin-Releasing Peptide Receptor (GRP-R) in Cancer Therapy: Development of Bombesin-Based Peptide-Drug Conjugates.Int J Mol Sci. 2023 Feb 8;24(4):3400. doi: 10.3390/ijms24043400. Int J Mol Sci. 2023. PMID: 36834815 Free PMC article.
-
PhAc-ALGP-Dox, a Novel Anticancer Prodrug with Targeted Activation and Improved Therapeutic Index.Mol Cancer Ther. 2022 Apr 1;21(4):568-581. doi: 10.1158/1535-7163.MCT-21-0518. Mol Cancer Ther. 2022. PMID: 35149549 Free PMC article.
References
-
- Joubert N., Denevault-Sabourin C., Bryden F., Viaud-Massuard M.C. Towards antibody-drug conjugates and prodrug strategies with extracellular stimuli-responsive drug delivery in the tumor microenvironment for cancer therapy. Eur. J. Med. Chem. 2017;142:393–415. doi: 10.1016/j.ejmech.2017.08.049. - DOI - PubMed
-
- Beck A., Terral G., Debaene F., Wagner-Rousset E., Marcoux J., Janin-Bussat M.-C., Colas O., Van Dorsselaer A., Cianférani S. Cutting-edge mass spectrometry methods for the multi-level structural characterization of antibody-drug conjugates. Expert Rev. Proteom. 2016;13:157–183. doi: 10.1586/14789450.2016.1132167. - DOI - PubMed
Publication types
Grants and funding
LinkOut - more resources
Full Text Sources
Other Literature Sources