A decade of research on the second messenger c-di-AMP
- PMID: 32472931
- PMCID: PMC7850090
- DOI: 10.1093/femsre/fuaa019
A decade of research on the second messenger c-di-AMP
Abstract
Cyclic dimeric adenosine 3',5'-monophosphate (c-di-AMP) is an emerging second messenger in bacteria and archaea that is synthesized from two molecules of ATP by diadenylate cyclases and degraded to pApA or two AMP molecules by c-di-AMP-specific phosphodiesterases. Through binding to specific protein- and riboswitch-type receptors, c-di-AMP regulates a wide variety of prokaryotic physiological functions, including maintaining the osmotic pressure, balancing central metabolism, monitoring DNA damage and controlling biofilm formation and sporulation. It mediates bacterial adaptation to a variety of environmental parameters and can also induce an immune response in host animal cells. In this review, we discuss the phylogenetic distribution of c-di-AMP-related enzymes and receptors and provide some insights into the various aspects of c-di-AMP signaling pathways based on more than a decade of research. We emphasize the key role of c-di-AMP in maintaining bacterial osmotic balance, especially in Gram-positive bacteria. In addition, we discuss the future direction and trends of c-di-AMP regulatory network, such as the likely existence of potential c-di-AMP transporter(s), the possibility of crosstalk between c-di-AMP signaling with other regulatory systems, and the effects of c-di-AMP compartmentalization. This review aims to cover the broad spectrum of research on the regulatory functions of c-di-AMP and c-di-AMP signaling pathways.
Keywords: c-di-AMP; metabolic enzyme; osmotic balance; physiological functions; protein receptor; riboswitch.
© FEMS 2020.
Figures
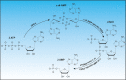
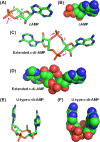
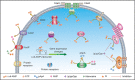
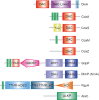
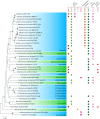
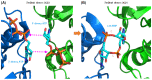
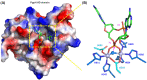
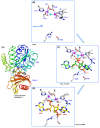
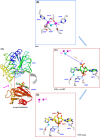
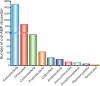
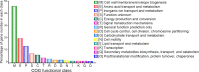
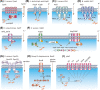
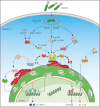
Similar articles
-
All DACs in a Row: Domain Architectures of Bacterial and Archaeal Diadenylate Cyclases.J Bacteriol. 2023 Apr 25;205(4):e0002323. doi: 10.1128/jb.00023-23. Epub 2023 Apr 6. J Bacteriol. 2023. PMID: 37022175 Free PMC article. Review.
-
A jack of all trades: the multiple roles of the unique essential second messenger cyclic di-AMP.Mol Microbiol. 2015 Jul;97(2):189-204. doi: 10.1111/mmi.13026. Epub 2015 May 9. Mol Microbiol. 2015. PMID: 25869574 Review.
-
Making and Breaking of an Essential Poison: the Cyclases and Phosphodiesterases That Produce and Degrade the Essential Second Messenger Cyclic di-AMP in Bacteria.J Bacteriol. 2018 Dec 7;201(1):e00462-18. doi: 10.1128/JB.00462-18. Print 2019 Jan 1. J Bacteriol. 2018. PMID: 30224435 Free PMC article. Review.
-
Cyclic di-AMP, a second messenger of primary importance: tertiary structures and binding mechanisms.Nucleic Acids Res. 2020 Apr 6;48(6):2807-2829. doi: 10.1093/nar/gkaa112. Nucleic Acids Res. 2020. PMID: 32095817 Free PMC article. Review.
-
[Advances in Research on the Role of Bacterial c-di-AMP in Host Immunity].Sichuan Da Xue Xue Bao Yi Xue Ban. 2022 Nov;53(6):1098-1103. doi: 10.12182/20220860102. Sichuan Da Xue Xue Bao Yi Xue Ban. 2022. PMID: 36443059 Free PMC article. Review. Chinese.
Cited by
-
Comprehensive discovery of novel structured noncoding RNAs in 26 bacterial genomes.RNA Biol. 2021 Dec;18(12):2417-2432. doi: 10.1080/15476286.2021.1917891. Epub 2021 May 10. RNA Biol. 2021. PMID: 33970790 Free PMC article.
-
All DACs in a Row: Domain Architectures of Bacterial and Archaeal Diadenylate Cyclases.J Bacteriol. 2023 Apr 25;205(4):e0002323. doi: 10.1128/jb.00023-23. Epub 2023 Apr 6. J Bacteriol. 2023. PMID: 37022175 Free PMC article. Review.
-
Open Issues for Protein Function Assignment in Haloferax volcanii and Other Halophilic Archaea.Genes (Basel). 2021 Jun 24;12(7):963. doi: 10.3390/genes12070963. Genes (Basel). 2021. PMID: 34202810 Free PMC article.
-
The Borrelia burgdorferi Adenylate Cyclase, CyaB, Is Important for Virulence Factor Production and Mammalian Infection.Front Microbiol. 2021 May 25;12:676192. doi: 10.3389/fmicb.2021.676192. eCollection 2021. Front Microbiol. 2021. PMID: 34113333 Free PMC article.
-
Fighting Tuberculosis: In Search of a BCG Replacement.Microorganisms. 2022 Dec 23;11(1):51. doi: 10.3390/microorganisms11010051. Microorganisms. 2022. PMID: 36677343 Free PMC article. Review.
References
Publication types
MeSH terms
Substances
LinkOut - more resources
Full Text Sources
Molecular Biology Databases