Stress-Responsive Alternative Sigma Factor SigB Plays a Positive Role in the Antifungal Proficiency of Bacillus subtilis
- PMID: 30824454
- PMCID: PMC6495766
- DOI: 10.1128/AEM.00178-19
Stress-Responsive Alternative Sigma Factor SigB Plays a Positive Role in the Antifungal Proficiency of Bacillus subtilis
Abstract
Different Bacillus species with PGPR (plant growth-promoting rhizobacterium) activity produce potent biofungicides and stimulate plant defense responses against phytopathogenic fungi. However, very little is known about how these PGPRs recognize phytopathogens and exhibit the antifungal response. Here, we report the antagonistic interaction between Bacillus subtilis and the phytopathogenic fungus Fusarium verticillioides We demonstrate that this bacterial-fungal interaction triggers the induction of the SigB transcription factor, the master regulator of B. subtilis stress adaptation. Dual-growth experiments performed with live or dead mycelia or culture supernatants of F. verticillioides showed that SigB was activated and required for the biocontrol of fungal growth. Mutations in the different regulatory pathways of SigB activation in the isogenic background revealed that only the energy-related RsbP-dependent arm of SigB activation was responsible for specific fungal detection and triggering the antagonistic response. The activation of SigB increased the expression of the operon responsible for the production of the antimicrobial cyclic lipopeptide surfactin (the srfA operon). SigB-deficient B. subtilis cultures produced decreased amounts of surfactin, and B. subtilis cultures defective in surfactin production (ΔsrfA) were unable to control the growth of F. verticillioidesIn vivo experiments of seed germination efficiency and early plant growth inhibition in the presence of F. verticillioides confirmed the physiological importance of SigB activity for plant bioprotection.IMPORTANCE Biological control using beneficial bacteria (PGPRs) represents an attractive and environment-friendly alternative to pesticides for controlling plant diseases. Different PGPR Bacillus species produce potent biofungicides and stimulate plant defense responses against phytopathogenic fungi. However, very little is known about how PGPRs recognize phytopathogens and process the antifungal response. Here, we report how B. subtilis triggers the induction of the stress-responsive sigma B transcription factor and the synthesis of the lipopeptide surfactin to fight the phytopathogen. Our findings show the participation of the stress-responsive regulon of PGPR Bacillus in the detection and biocontrol of a phytopathogenic fungus of agronomic impact.
Keywords: Bacillus subtilis; bicontrol; fungi; sigma B; stress; surfactin.
Copyright © 2019 American Society for Microbiology.
Figures
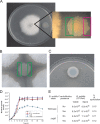
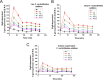
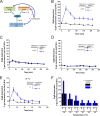
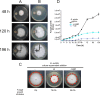
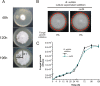
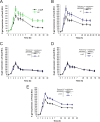
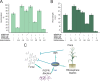
Similar articles
-
The Stress-Responsive Alternative Sigma Factor SigB of Bacillus subtilis and Its Relatives: An Old Friend With New Functions.Front Microbiol. 2020 Sep 15;11:1761. doi: 10.3389/fmicb.2020.01761. eCollection 2020. Front Microbiol. 2020. PMID: 33042030 Free PMC article. Review.
-
Lipopeptide mediated biocontrol activity of endophytic Bacillus subtilis against fungal phytopathogens.BMC Microbiol. 2019 Apr 2;19(1):71. doi: 10.1186/s12866-019-1440-8. BMC Microbiol. 2019. PMID: 30940070 Free PMC article.
-
Chill induction of the SigB-dependent general stress response in Bacillus subtilis and its contribution to low-temperature adaptation.J Bacteriol. 2003 Aug;185(15):4305-14. doi: 10.1128/JB.185.15.4305-4314.2003. J Bacteriol. 2003. PMID: 12867438 Free PMC article.
-
Regulation of Biofilm Aging and Dispersal in Bacillus subtilis by the Alternative Sigma Factor SigB.J Bacteriol. 2018 Dec 20;201(2):e00473-18. doi: 10.1128/JB.00473-18. Print 2019 Jan 15. J Bacteriol. 2018. PMID: 30396900 Free PMC article.
-
Non-specific, general and multiple stress resistance of growth-restricted Bacillus subtilis cells by the expression of the sigmaB regulon.Mol Microbiol. 1998 Sep;29(5):1129-36. doi: 10.1046/j.1365-2958.1998.00977.x. Mol Microbiol. 1998. PMID: 9767581 Review.
Cited by
-
SigB modulates expression of novel SigB regulon members via Bc1009 in non-stressed and heat-stressed cells revealing its alternative roles in Bacillus cereus.BMC Microbiol. 2023 Feb 10;23(1):37. doi: 10.1186/s12866-023-02783-3. BMC Microbiol. 2023. PMID: 36759782 Free PMC article.
-
Surfactin Shows Relatively Low Antimicrobial Activity against Bacillus subtilis and Other Bacterial Model Organisms in the Absence of Synergistic Metabolites.Microorganisms. 2022 Apr 5;10(4):779. doi: 10.3390/microorganisms10040779. Microorganisms. 2022. PMID: 35456828 Free PMC article.
-
A Newly Isolated Bacillus subtilis Strain Named WS-1 Inhibited Diarrhea and Death Caused by Pathogenic Escherichia coli in Newborn Piglets.Front Microbiol. 2019 Jun 12;10:1248. doi: 10.3389/fmicb.2019.01248. eCollection 2019. Front Microbiol. 2019. PMID: 31249559 Free PMC article.
-
Antifungal effect and some properties of cell-free supernatants of two Bacillus subtilis isolates against Fusarium verticillioides.Braz J Microbiol. 2024 Sep;55(3):2527-2538. doi: 10.1007/s42770-024-01414-x. Epub 2024 Jun 11. Braz J Microbiol. 2024. PMID: 38862737
-
The Stress-Responsive Alternative Sigma Factor SigB of Bacillus subtilis and Its Relatives: An Old Friend With New Functions.Front Microbiol. 2020 Sep 15;11:1761. doi: 10.3389/fmicb.2020.01761. eCollection 2020. Front Microbiol. 2020. PMID: 33042030 Free PMC article. Review.
References
Publication types
MeSH terms
Substances
LinkOut - more resources
Full Text Sources
Molecular Biology Databases