Regulation of Biofilm Aging and Dispersal in Bacillus subtilis by the Alternative Sigma Factor SigB
- PMID: 30396900
- PMCID: PMC6304664
- DOI: 10.1128/JB.00473-18
Regulation of Biofilm Aging and Dispersal in Bacillus subtilis by the Alternative Sigma Factor SigB
Abstract
Bacterial biofilms are important in natural settings, biotechnology, and medicine. However, regulation of biofilm development and its persistence in different niches is complex and only partially understood. One key step during the biofilm life cycle is dispersal, when motile cells abandon the mature biofilm to spread out and colonize new niches. Here, we show that in the model bacterium Bacillus subtilis the general stress transcription factor SigB is essential for halting detrimental overgrowth of mature biofilm and for triggering dispersal when nutrients become limited. Specifically, SigB-deficient biofilms were larger than wild-type biofilms but exhibited accelerated cell death, significantly greater sensitivity to different stresses, and reduced dispersal. Interestingly, the signal detected by SigB to limit biofilm growth was transduced through the RsbP-dependent metabolic arm of the SigB regulatory cascade, which in turn positively controlled expression of SinR, the master regulator of biofilm formation and cell motility. This novel SigB-SinR regulatory circuit might be important in controlling the fitness of biofilms (either beneficial or harmful) in diverse environments.IMPORTANCE Biofilms are crucial for bacterial survival, adaptation, and dissemination in natural, industrial, and medical systems. Sessile cells embedded in the self-produced extracellular matrix of the biofilm benefit from a division of labor and are protected from environmental insults. However, as the biofilm ages, cells become stressed because of overcrowding, starvation, and accumulation of waste products. How does the sessile biofilm community sense and respond to stressful conditions? Here, we show that in Bacillus subtilis, the transcription factors SigB and SinR control whether cells remain in or leave a biofilm when metabolic conditions become unfavorable. This novel SigB-SinR regulatory circuit might be important for controlling the fitness of biofilms (either beneficial or harmful) in diverse environments.
Keywords: Bacillus subtilis; biofilm aging; biofilm dispersal; sigma B; stress activation.
Copyright © 2018 American Society for Microbiology.
Figures
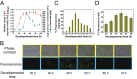
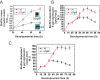
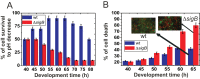
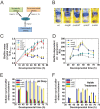
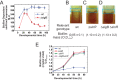
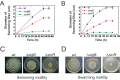
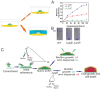
Similar articles
-
The Stress-Responsive Alternative Sigma Factor SigB of Bacillus subtilis and Its Relatives: An Old Friend With New Functions.Front Microbiol. 2020 Sep 15;11:1761. doi: 10.3389/fmicb.2020.01761. eCollection 2020. Front Microbiol. 2020. PMID: 33042030 Free PMC article. Review.
-
Stress-Responsive Alternative Sigma Factor SigB Plays a Positive Role in the Antifungal Proficiency of Bacillus subtilis.Appl Environ Microbiol. 2019 Apr 18;85(9):e00178-19. doi: 10.1128/AEM.00178-19. Print 2019 May 1. Appl Environ Microbiol. 2019. PMID: 30824454 Free PMC article.
-
Selective Pressure for Biofilm Formation in Bacillus subtilis: Differential Effect of Mutations in the Master Regulator SinR on Bistability.mBio. 2018 Sep 4;9(5):e01464-18. doi: 10.1128/mBio.01464-18. mBio. 2018. PMID: 30181249 Free PMC article.
-
RsbV-independent induction of the SigB-dependent general stress regulon of Bacillus subtilis during growth at high temperature.J Bacteriol. 2004 Sep;186(18):6150-8. doi: 10.1128/JB.186.18.6150-6158.2004. J Bacteriol. 2004. PMID: 15342585 Free PMC article.
-
Bacillus subtilis biofilm formation and social interactions.Nat Rev Microbiol. 2021 Sep;19(9):600-614. doi: 10.1038/s41579-021-00540-9. Epub 2021 Apr 6. Nat Rev Microbiol. 2021. PMID: 33824496 Review.
Cited by
-
Novel prokaryotic system employing previously unknown nucleic acids-based receptors.Microb Cell Fact. 2022 Oct 4;21(1):202. doi: 10.1186/s12934-022-01923-0. Microb Cell Fact. 2022. PMID: 36195904 Free PMC article.
-
A Crosstalk Between Brachypodium Root Exudates, Organic Acids, and Bacillus velezensis B26, a Growth Promoting Bacterium.Front Microbiol. 2020 Oct 6;11:575578. doi: 10.3389/fmicb.2020.575578. eCollection 2020. Front Microbiol. 2020. PMID: 33123106 Free PMC article.
-
6S-2 RNA deletion in the undomesticated B. subtilis strain NCIB 3610 causes a biofilm derepression phenotype.RNA Biol. 2021 Jan;18(1):79-92. doi: 10.1080/15476286.2020.1795408. Epub 2020 Aug 30. RNA Biol. 2021. PMID: 32862759 Free PMC article.
-
SigB modulates expression of novel SigB regulon members via Bc1009 in non-stressed and heat-stressed cells revealing its alternative roles in Bacillus cereus.BMC Microbiol. 2023 Feb 10;23(1):37. doi: 10.1186/s12866-023-02783-3. BMC Microbiol. 2023. PMID: 36759782 Free PMC article.
-
Prediction and validation of novel SigB regulon members in Bacillus subtilis and regulon structure comparison to Bacillales members.BMC Microbiol. 2023 Jan 18;23(1):17. doi: 10.1186/s12866-022-02700-0. BMC Microbiol. 2023. PMID: 36653740 Free PMC article.
References
Publication types
MeSH terms
Substances
LinkOut - more resources
Full Text Sources
Other Literature Sources
Molecular Biology Databases