More than Enzymes That Make or Break Cyclic Di-GMP-Local Signaling in the Interactome of GGDEF/EAL Domain Proteins of Escherichia coli
- PMID: 29018125
- PMCID: PMC5635695
- DOI: 10.1128/mBio.01639-17
More than Enzymes That Make or Break Cyclic Di-GMP-Local Signaling in the Interactome of GGDEF/EAL Domain Proteins of Escherichia coli
Abstract
The bacterial second messenger bis-(3'-5')-cyclic diguanosine monophosphate (c-di-GMP) ubiquitously promotes bacterial biofilm formation. Intracellular pools of c-di-GMP seem to be dynamically negotiated by diguanylate cyclases (DGCs, with GGDEF domains) and specific phosphodiesterases (PDEs, with EAL or HD-GYP domains). Most bacterial species possess multiple DGCs and PDEs, often with surprisingly distinct and specific output functions. One explanation for such specificity is "local" c-di-GMP signaling, which is believed to involve direct interactions between specific DGC/PDE pairs and c-di-GMP-binding effector/target systems. Here we present a systematic analysis of direct protein interactions among all 29 GGDEF/EAL domain proteins of Escherichia coli Since the effects of interactions depend on coexpression and stoichiometries, cellular levels of all GGDEF/EAL domain proteins were also quantified and found to vary dynamically along the growth cycle. Instead of detecting specific pairs of interacting DGCs and PDEs, we discovered a tightly interconnected protein network of a specific subset or "supermodule" of DGCs and PDEs with a coregulated core of five hyperconnected hub proteins. These include the DGC/PDE proteins representing the c-di-GMP switch that turns on biofilm matrix production in E. coli Mutants lacking these core hub proteins show drastic biofilm-related phenotypes but no changes in cellular c-di-GMP levels. Overall, our results provide the basis for a novel model of local c-di-GMP signaling in which a single strongly expressed master PDE, PdeH, dynamically eradicates global effects of several DGCs by strongly draining the global c-di-GMP pool and thereby restricting these DGCs to serving as local c-di-GMP sources that activate specific colocalized effector/target systems.IMPORTANCE c-di-GMP signaling in bacteria is believed to occur via changes in cellular c-di-GMP levels controlled by antagonistic and potentially interacting pairs of diguanylate cyclases (DGCs) and c-di-GMP phosphodiesterases (PDEs). Our systematic analysis of protein-protein interaction patterns of all 29 GGDEF/EAL domain proteins of E. coli, together with our measurements of cellular c-di-GMP levels, challenges both aspects of this current concept. Knocking out distinct DGCs and PDEs has drastic effects on E. coli biofilm formation without changing the cellular c-di-GMP level. In addition, rather than generally coming in interacting DGC/PDE pairs, a subset of DGCs and PDEs operates as central interaction hubs in a larger "supermodule," with other DGCs and PDEs behaving as "lonely players" without contacts to other c-di-GMP-related enzymes. On the basis of these data, we propose a novel concept of "local" c-di-GMP signaling in bacteria with multiple enzymes that make or break the second messenger c-di-GMP.
Keywords: biofilms; c-di-GMP; cellulose; curli; diguanylate cyclase; second messenger.
Copyright © 2017 Sarenko et al.
Figures
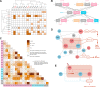
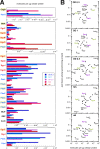
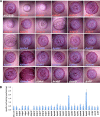
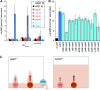
Similar articles
-
Genome-Based Comparison of Cyclic Di-GMP Signaling in Pathogenic and Commensal Escherichia coli Strains.J Bacteriol. 2015 Aug 24;198(1):111-26. doi: 10.1128/JB.00520-15. Print 2016 Jan 1. J Bacteriol. 2015. PMID: 26303830 Free PMC article.
-
Systematic Nomenclature for GGDEF and EAL Domain-Containing Cyclic Di-GMP Turnover Proteins of Escherichia coli.J Bacteriol. 2015 Jul 6;198(1):7-11. doi: 10.1128/JB.00424-15. Print 2016 Jan 1. J Bacteriol. 2015. PMID: 26148715 Free PMC article.
-
Enzymatically active and inactive phosphodiesterases and diguanylate cyclases are involved in regulation of Motility or sessility in Escherichia coli CFT073.mBio. 2012 Oct 9;3(5):e00307-12. doi: 10.1128/mBio.00307-12. mBio. 2012. PMID: 23047748 Free PMC article.
-
Trigger phosphodiesterases as a novel class of c-di-GMP effector proteins.Philos Trans R Soc Lond B Biol Sci. 2016 Nov 5;371(1707):20150498. doi: 10.1098/rstb.2015.0498. Philos Trans R Soc Lond B Biol Sci. 2016. PMID: 27672149 Free PMC article. Review.
-
Diguanylate Cyclases in Vibrio cholerae: Essential Regulators of Lifestyle Switching.Front Cell Infect Microbiol. 2020 Oct 22;10:582947. doi: 10.3389/fcimb.2020.582947. eCollection 2020. Front Cell Infect Microbiol. 2020. PMID: 33194821 Free PMC article. Review.
Cited by
-
The Wsp chemosensory system modulates c-di-GMP-dependent biofilm formation by integrating DSF quorum sensing through the WspR-RpfG complex in Lysobacter.NPJ Biofilms Microbiomes. 2022 Dec 16;8(1):97. doi: 10.1038/s41522-022-00365-1. NPJ Biofilms Microbiomes. 2022. PMID: 36526637 Free PMC article.
-
Differential Regulation of Genes for Cyclic-di-GMP Metabolism Orchestrates Adaptive Changes During Rhizosphere Colonization by Pseudomonas fluorescens.Front Microbiol. 2019 May 16;10:1089. doi: 10.3389/fmicb.2019.01089. eCollection 2019. Front Microbiol. 2019. PMID: 31156596 Free PMC article.
-
Dissecting Light Sensing and Metabolic Pathways on the Millimeter Scale in High-Altitude Modern Stromatolites.Microb Ecol. 2023 Aug;86(2):914-932. doi: 10.1007/s00248-022-02112-7. Epub 2022 Sep 26. Microb Ecol. 2023. PMID: 36161499
-
Nucleotide signaling pathway convergence in a cAMP-sensing bacterial c-di-GMP phosphodiesterase.EMBO J. 2019 Sep 2;38(17):e100772. doi: 10.15252/embj.2018100772. Epub 2019 Jul 29. EMBO J. 2019. PMID: 31355487 Free PMC article.
-
Comparative Genomics of Cyclic di-GMP Metabolism and Chemosensory Pathways in Shewanella algae Strains: Novel Bacterial Sensory Domains and Functional Insights into Lifestyle Regulation.mSystems. 2022 Apr 26;7(2):e0151821. doi: 10.1128/msystems.01518-21. Epub 2022 Mar 21. mSystems. 2022. PMID: 35311563 Free PMC article.
References
MeSH terms
Substances
LinkOut - more resources
Full Text Sources
Other Literature Sources
Molecular Biology Databases
Miscellaneous