Maturation of a recurrent excitatory neocortical circuit by experience-dependent unsilencing of newly formed dendritic spines
- PMID: 21555076
- PMCID: PMC3092126
- DOI: 10.1016/j.neuron.2011.02.057
Maturation of a recurrent excitatory neocortical circuit by experience-dependent unsilencing of newly formed dendritic spines
Erratum in
- Neuron. 2011 Jun 9;70(5):1020
Abstract
Local recurrent excitatory circuits are ubiquitous in neocortex, yet little is known about their development or architecture. Here we introduce a quantitative technique for efficient single-cell resolution circuit mapping using 2-photon (2P) glutamate uncaging and analyze experience-dependent neonatal development of the layer 4 barrel cortex local excitatory circuit. We show that sensory experience specifically drives a 3-fold increase in connectivity at postnatal day (P) 9, producing a highly recurrent network. A profound dendritic spinogenesis occurs concurrent with the connectivity increase, but this is not experience dependent. However, in experience-deprived cortex, a much greater proportion of spines lack postsynaptic AMPA receptors (AMPARs) and synaptic connectivity via NMDA receptors (NMDARs) is the same as in normally developing cortex. Thus we describe a approach for quantitative circuit mapping and show that sensory experience sculpts an intrinsically developing template network, which is based on NMDAR-only synapses, by driving AMPARs into newly formed silent spines.
Copyright © 2011 Elsevier Inc. All rights reserved.
Figures
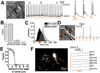
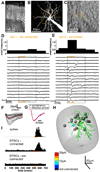
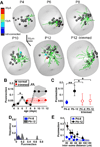
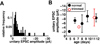
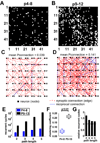
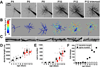
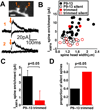
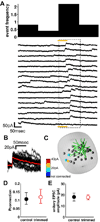
Similar articles
-
mGluR5 Exerts Cell-Autonomous Influences on the Functional and Anatomical Development of Layer IV Cortical Neurons in the Mouse Primary Somatosensory Cortex.J Neurosci. 2016 Aug 24;36(34):8802-14. doi: 10.1523/JNEUROSCI.1224-16.2016. J Neurosci. 2016. PMID: 27559164 Free PMC article.
-
Experience-dependent increase in spine calcium evoked by backpropagating action potentials in layer 2/3 pyramidal neurons in rat somatosensory cortex.Eur J Neurosci. 2009 Nov;30(10):1870-7. doi: 10.1111/j.1460-9568.2009.06977.x. Epub 2009 Nov 11. Eur J Neurosci. 2009. PMID: 19912332
-
Emergence of cortical inhibition by coordinated sensory-driven plasticity at distinct synaptic loci.Nat Neurosci. 2010 Oct;13(10):1240-8. doi: 10.1038/nn.2639. Nat Neurosci. 2010. PMID: 20871602 Free PMC article.
-
Developmental switch in spike timing-dependent plasticity at layers 4-2/3 in the rodent barrel cortex.J Neurosci. 2012 Oct 24;32(43):15000-11. doi: 10.1523/JNEUROSCI.2506-12.2012. J Neurosci. 2012. PMID: 23100422 Free PMC article.
-
Synaptic structure and function in the mouse somatosensory cortex during chronic pain: in vivo two-photon imaging.Neural Plast. 2012;2012:640259. doi: 10.1155/2012/640259. Epub 2012 Feb 22. Neural Plast. 2012. PMID: 22530157 Free PMC article. Review.
Cited by
-
Progressive maturation of silent synapses governs the duration of a critical period.Proc Natl Acad Sci U S A. 2015 Jun 16;112(24):E3131-40. doi: 10.1073/pnas.1506488112. Epub 2015 May 26. Proc Natl Acad Sci U S A. 2015. PMID: 26015564 Free PMC article.
-
A role for silent synapses in the development of the pathway from layer 2/3 to 5 pyramidal cells in the neocortex.J Neurosci. 2012 Sep 19;32(38):13085-99. doi: 10.1523/JNEUROSCI.1262-12.2012. J Neurosci. 2012. PMID: 22993426 Free PMC article.
-
A databank for intracellular electrophysiological mapping of the adult somatosensory cortex.Gigascience. 2018 Dec 1;7(12):giy147. doi: 10.1093/gigascience/giy147. Gigascience. 2018. PMID: 30521020 Free PMC article.
-
An opposing function of paralogs in balancing developmental synapse maturation.PLoS Biol. 2018 Dec 26;16(12):e2006838. doi: 10.1371/journal.pbio.2006838. eCollection 2018 Dec. PLoS Biol. 2018. PMID: 30586380 Free PMC article.
-
Decreasing-Rate Pruning Optimizes the Construction of Efficient and Robust Distributed Networks.PLoS Comput Biol. 2015 Jul 28;11(7):e1004347. doi: 10.1371/journal.pcbi.1004347. eCollection 2015 Jul. PLoS Comput Biol. 2015. PMID: 26217933 Free PMC article.
References
-
- Allen CB, Celikel T, Feldman DE. Long-term depression induced by sensory deprivation during cortical map plasticity in vivo. Nat Neurosci. 2003;6:291–299. - PubMed
-
- Benshalom G, White EL. Quantification of thalamocortical synapses with spiny stellate neurons in layer IV of mouse somatosensory cortex. J Comp Neurol. 1986;253:303–314. - PubMed
-
- Bonifazi P, Goldin M, Picardo MA, Jorquera I, Cattani A, Bianconi G, Represa A, Ben-Ari Y, Cossart R. GABAergic hub neurons orchestrate synchrony in developing hippocampal networks. Science. 2009;326:1419–1424. - PubMed
-
- Braitenberg V, Schüz A. Cortex: Statistics and Geometry of Neuronal Connectivity. 2nd ed. Springer; 1998.
Publication types
MeSH terms
Substances
Grants and funding
LinkOut - more resources
Full Text Sources
Other Literature Sources