A missense mutation in CASK causes FG syndrome in an Italian family
- PMID: 19200522
- PMCID: PMC2668001
- DOI: 10.1016/j.ajhg.2008.12.018
A missense mutation in CASK causes FG syndrome in an Italian family
Abstract
First described in 1974, FG syndrome (FGS) is an X-linked multiple congenital anomaly/mental retardation (MCA/MR) disorder, characterized by high clinical variability and genetic heterogeneity. Five loci (FGS1-5) have so far been linked to this phenotype on the X chromosome, but only one gene, MED12, has been identified to date. Mutations in this gene account for a restricted number of FGS patients with a more distinctive phenotype, referred to as the Opitz-Kaveggia phenotype. We report here that a p.R28L (c.83G-->T) missense mutation in CASK causes FGS phenotype in an Italian family previously mapped to Xp11.4-p11.3 (FGS4). The identified missense mutation cosegregates with the phenotype in this family and is absent in 1000 control X chromosomes of the same ethnic origin. An extensive analysis of CASK protein functions as well as structural and dynamic studies performed by molecular dynamics (MD) simulation did not reveal significant alterations induced by the p.R28L substitution. However, we observed a partial skipping of the exon 2 of CASK, presumably a consequence of improper recognition of exonic splicing enhancers (ESEs) induced by the c.83G-->T transversion. CASK is a multidomain scaffold protein highly expressed in the central nervous system (CNS) with specific localization to the synapses, where it forms large signaling complexes regulating neurotransmission. We suggest that the observed phenotype is most likely a consequence of an altered CASK expression profile during embryogenesis, brain development, and differentiation.
Figures
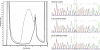
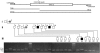
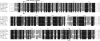
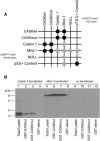
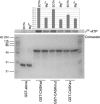
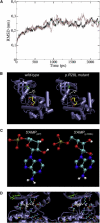
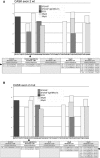
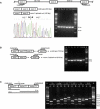
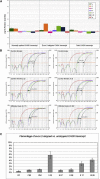
Similar articles
-
Genetic heterogeneity of FG syndrome: a fourth locus (FGS4) maps to Xp11.4-p11.3 in an Italian family.Hum Genet. 2003 Feb;112(2):124-30. doi: 10.1007/s00439-002-0863-7. Epub 2002 Nov 13. Hum Genet. 2003. PMID: 12522552
-
Clinical experience in the evaluation of 30 patients with a prior diagnosis of FG syndrome.J Med Genet. 2009 Jan;46(1):9-13. doi: 10.1136/jmg.2008.060509. Epub 2008 Sep 19. J Med Genet. 2009. PMID: 18805826
-
Clinical and neurocognitive characterization of a family with a novel MED12 gene frameshift mutation.Am J Med Genet A. 2013 Dec;161A(12):3063-71. doi: 10.1002/ajmg.a.36162. Epub 2013 Aug 16. Am J Med Genet A. 2013. PMID: 24039113
-
MED12 missense mutation in a three-generation family. Clinical characterization of MED12-related disorders and literature review.Eur J Med Genet. 2020 Mar;63(3):103768. doi: 10.1016/j.ejmg.2019.103768. Epub 2019 Sep 16. Eur J Med Genet. 2020. PMID: 31536828 Review.
-
Behavioral features in young adults with FG syndrome (Opitz-Kaveggia syndrome).Am J Med Genet C Semin Med Genet. 2010 Nov 15;154C(4):477-85. doi: 10.1002/ajmg.c.30284. Am J Med Genet C Semin Med Genet. 2010. PMID: 20981778 Free PMC article. Review.
Cited by
-
Interrogation and validation of the interactome of neuronal Munc18-interacting Mint proteins with AlphaFold2.J Biol Chem. 2024 Jan;300(1):105541. doi: 10.1016/j.jbc.2023.105541. Epub 2023 Dec 9. J Biol Chem. 2024. PMID: 38072052 Free PMC article.
-
Alterations of presynaptic proteins in autism spectrum disorder.Front Mol Neurosci. 2022 Nov 17;15:1062878. doi: 10.3389/fnmol.2022.1062878. eCollection 2022. Front Mol Neurosci. 2022. PMID: 36466804 Free PMC article. Review.
-
Drosophila CASK regulates brain size and neuronal morphogenesis, providing a genetic model of postnatal microcephaly suitable for drug discovery.Neural Dev. 2023 Oct 7;18(1):6. doi: 10.1186/s13064-023-00174-y. Neural Dev. 2023. PMID: 37805506 Free PMC article.
-
Structural Analysis Implicates CASK-Liprin-α2 Interaction in Cerebellar Granular Cell Death in MICPCH Syndrome.Cells. 2023 Apr 18;12(8):1177. doi: 10.3390/cells12081177. Cells. 2023. PMID: 37190086 Free PMC article.
-
Calcium/calmodulin-dependent serine protein kinase (CASK), a protein implicated in mental retardation and autism-spectrum disorders, interacts with T-Brain-1 (TBR1) to control extinction of associative memory in male mice.J Psychiatry Neurosci. 2017 Jan;42(1):37-47. doi: 10.1503/jpn.150359. J Psychiatry Neurosci. 2017. PMID: 28234597 Free PMC article.
References
-
- Opitz J.M., Kaveggia E.G. Studies of malformation syndromes of man 33: the FG syndrome. An X- linked recessive syndrome of multiple congenital anomalies and mental retardation. Z. Kinderheilkd. 1974;117:1–18. - PubMed
-
- Opitz J.M., Richieri-da Costa A., Aase J.M., Benke P.J. FG syndrome update 1988: note of 5 new patients and bibliography. Am. J. Med. Genet. 1988;30:309–328. - PubMed
-
- Romano C., Baraitser M., Thompson E. A clinical follow-up of British patients with FG syndrome. Clin. Dysmorphol. 1994;3:104–114. - PubMed
-
- Thompson E.M., Baraitser M., Lindenbaum R.H., Zaidi Z.H., Kroll J.S. The FG syndrome: 7 new cases. Clin. Genet. 1985;27:582–594. - PubMed
-
- Neri G., Blumberg B., Miles P.V., Opitz J.M. Sensorineural deafness in the FG syndrome: report on four new cases. Am. J. Med. Genet. 1984;19:369–377. - PubMed
Publication types
MeSH terms
Substances
Grants and funding
LinkOut - more resources
Full Text Sources
Molecular Biology Databases