Aspm specifically maintains symmetric proliferative divisions of neuroepithelial cells
- PMID: 16798874
- PMCID: PMC1502476
- DOI: 10.1073/pnas.0604066103
Aspm specifically maintains symmetric proliferative divisions of neuroepithelial cells
Abstract
The ASPM (abnormal spindle-like microcephaly-associated) protein has previously been implicated in the determination of human cerebral cortical size, but the cell biological basis of this regulation has not been studied. Here we investigate the role of Aspm in mouse embryonic neuroepithelial (NE) cells, the primary stem and progenitor cells of the mammalian brain. Aspm was found to be concentrated at mitotic spindle poles of NE cells and to be down-regulated with their switch from proliferative to neurogenic divisions. Upon RNA interference in telencephalic NE cells, Aspm mRNA is reduced, mitotic spindle poles lack Aspm protein, and the cleavage plane of NE cells is less frequently oriented perpendicular to the ventricular surface of the neuroepithelium. The alteration in the cleavage plane orientation of NE cells increases the probability that these highly polarized cells undergo asymmetric division, i.e., that apical plasma membrane is inherited by only one of the daughter cells. Concomitant with the resulting increase in abventricular cells in the ventricular zone, a larger proportion of NE cell progeny is found in the neuronal layer, implying a reduction in the number of NE progenitor cells upon Aspm knock-down relative to control. Our results demonstrate that Aspm is crucial for maintaining a cleavage plane orientation that allows symmetric, proliferative divisions of NE cells during brain development. These data provide a cell biological explanation of the primary microcephaly observed in humans with mutations in ASPM, which also has implications for the evolution of mammalian brains.
Conflict of interest statement
Conflict of interest statement: No conflicts declared.
Figures
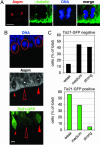
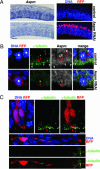
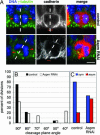
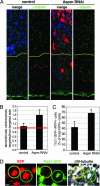
Similar articles
-
ASPM and CITK regulate spindle orientation by affecting the dynamics of astral microtubules.EMBO Rep. 2016 Oct;17(10):1396-1409. doi: 10.15252/embr.201541823. Epub 2016 Aug 25. EMBO Rep. 2016. PMID: 27562601 Free PMC article.
-
Asymmetric distribution of the apical plasma membrane during neurogenic divisions of mammalian neuroepithelial cells.EMBO J. 2004 Jun 2;23(11):2314-24. doi: 10.1038/sj.emboj.7600223. Epub 2004 May 13. EMBO J. 2004. PMID: 15141162 Free PMC article.
-
Human ASPM participates in spindle organisation, spindle orientation and cytokinesis.BMC Cell Biol. 2010 Nov 2;11:85. doi: 10.1186/1471-2121-11-85. BMC Cell Biol. 2010. PMID: 21044324 Free PMC article.
-
The Multiple Mitotic Roles of the ASPM Orthologous Proteins: Insight into the Etiology of ASPM-Dependent Microcephaly.Cells. 2023 Mar 16;12(6):922. doi: 10.3390/cells12060922. Cells. 2023. PMID: 36980263 Free PMC article. Review.
-
LIS1 and spindle orientation in neuroepithelial cells.Cell Stem Cell. 2008 Mar 6;2(3):193-4. doi: 10.1016/j.stem.2008.02.010. Cell Stem Cell. 2008. PMID: 18371441 Review.
Cited by
-
Whole Exome Sequencing Identifies Three Novel Mutations in the ASPM Gene From Saudi Families Leading to Primary Microcephaly.Front Pediatr. 2021 Feb 11;8:627122. doi: 10.3389/fped.2020.627122. eCollection 2020. Front Pediatr. 2021. PMID: 33643967 Free PMC article.
-
New insights into the mechanism of dynein motor regulation by lissencephaly-1.Elife. 2020 Jul 21;9:e59737. doi: 10.7554/eLife.59737. Elife. 2020. PMID: 32692650 Free PMC article.
-
Aberrant ASPM expression mediated by transcriptional regulation of FoxM1 promotes the progression of gliomas.J Cell Mol Med. 2020 Sep;24(17):9613-9626. doi: 10.1111/jcmm.15435. Epub 2020 Jul 15. J Cell Mol Med. 2020. PMID: 32667745 Free PMC article.
-
Nrf2/ASPM axis regulated vasculogenic mimicry formation in hepatocellular carcinoma under hypoxia.J Gastroenterol. 2024 Oct;59(10):941-957. doi: 10.1007/s00535-024-02140-9. Epub 2024 Aug 3. J Gastroenterol. 2024. PMID: 39097533
-
Regulation of mitotic spindle orientation: an integrated view.EMBO Rep. 2016 Aug;17(8):1106-30. doi: 10.15252/embr.201642292. Epub 2016 Jul 18. EMBO Rep. 2016. PMID: 27432284 Free PMC article. Review.
References
Publication types
MeSH terms
Substances
LinkOut - more resources
Full Text Sources
Medical
Molecular Biology Databases