Mutual interactions between subunits of the human RNase MRP ribonucleoprotein complex
- PMID: 15096576
- PMCID: PMC407822
- DOI: 10.1093/nar/gkh539
Mutual interactions between subunits of the human RNase MRP ribonucleoprotein complex
Abstract
The eukaryotic ribonuclease for mitochondrial RNA processing (RNase MRP) is mainly located in the nucleoli and belongs to the small nucleolar ribonucleoprotein (snoRNP) particles. RNase MRP is involved in the processing of pre-rRNA and the generation of RNA primers for mitochondrial DNA replication. A closely related snoRNP, which shares protein subunits with RNase MRP and contains a structurally related RNA subunit, is the pre-tRNA processing factor RNase P. Up to now, 10 protein subunits of these complexes have been described, designated hPop1, hPop4, hPop5, Rpp14, Rpp20, Rpp21, Rpp25, Rpp30, Rpp38 and Rpp40. To get more insight into the assembly of the human RNase MRP complex we studied protein-protein and protein-RNA interactions by means of GST pull-down experiments. A total of 19 direct protein-protein and six direct protein-RNA interactions were observed. The analysis of mutant RNase MRP RNAs showed that distinct regions are involved in the direct interaction with protein subunits. The results provide insight into the way the protein and RNA subunits assemble into a ribonucleoprotein particle. Based upon these data a new model for the architecture of the human RNase MRP complex was generated.
Figures
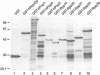
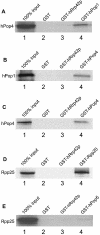
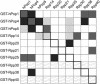
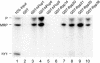
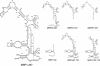
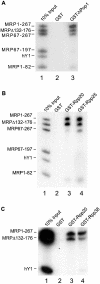
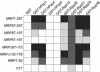
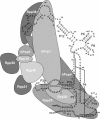
Similar articles
-
Differential association of protein subunits with the human RNase MRP and RNase P complexes.RNA. 2006 Jul;12(7):1373-82. doi: 10.1261/rna.2293906. Epub 2006 May 24. RNA. 2006. PMID: 16723659 Free PMC article.
-
Probing the structure of Saccharomyces cerevisiae RNase MRP.Biochem Soc Trans. 2005 Jun;33(Pt 3):479-81. doi: 10.1042/BST0330479. Biochem Soc Trans. 2005. PMID: 15916546
-
Architecture and function of the human endonucleases RNase P and RNase MRP.IUBMB Life. 2000 Apr;49(4):265-72. doi: 10.1080/15216540050033113. IUBMB Life. 2000. PMID: 10995027 Review.
-
A conserved element in the yeast RNase MRP RNA subunit can participate in a long-range base-pairing interaction.J Mol Biol. 2004 Aug 6;341(2):375-88. doi: 10.1016/j.jmb.2004.05.076. J Mol Biol. 2004. PMID: 15276830
-
RNase MRP RNA and human genetic diseases.Cell Res. 2007 Mar;17(3):219-26. doi: 10.1038/sj.cr.7310120. Cell Res. 2007. PMID: 17189938 Review.
Cited by
-
New insights into the role of ribonuclease P protein subunit p30 from tumor to internal reference.Front Oncol. 2022 Oct 13;12:1018279. doi: 10.3389/fonc.2022.1018279. eCollection 2022. Front Oncol. 2022. PMID: 36313673 Free PMC article. Review.
-
Unexpected diversity of RNase P, an ancient tRNA processing enzyme: challenges and prospects.FEBS Lett. 2010 Jan 21;584(2):287-96. doi: 10.1016/j.febslet.2009.11.048. FEBS Lett. 2010. PMID: 19931535 Free PMC article. Review.
-
Predicting protein associations with long noncoding RNAs.Nat Methods. 2011 Jun;8(6):444-5. doi: 10.1038/nmeth.1611. Nat Methods. 2011. PMID: 21623348 No abstract available.
-
Whole-exome re-sequencing in a family quartet identifies POP1 mutations as the cause of a novel skeletal dysplasia.PLoS Genet. 2011 Mar;7(3):e1002027. doi: 10.1371/journal.pgen.1002027. Epub 2011 Mar 24. PLoS Genet. 2011. PMID: 21455487 Free PMC article.
-
Clinical and molecular diagnosis of a cartilage-hair hypoplasia with IGF-1 deficiency.Am J Med Genet A. 2017 Feb;173(2):537-540. doi: 10.1002/ajmg.a.38052. Epub 2016 Nov 14. Am J Med Genet A. 2017. PMID: 27862957 Free PMC article.
References
-
- Lygerou Z., Allmang,C., Tollervey,D. and Seraphin,B. (1996) Accurate processing of a eukaryotic precursor ribosomal RNA by ribonuclease MRP in vitro.Science, 272, 268–270. - PubMed
-
- Chang D.D. and Clayton,D.A. (1987) A mammalian mitochondrial RNA processing activity contains nucleus-encoded RNA. Science, 235, 1178–1184. - PubMed
Publication types
MeSH terms
Substances
LinkOut - more resources
Full Text Sources
Molecular Biology Databases
Research Materials