The role of the TIM8-13 complex in the import of Tim23 into mitochondria
- PMID: 11101512
- PMCID: PMC305865
- DOI: 10.1093/emboj/19.23.6392
The role of the TIM8-13 complex in the import of Tim23 into mitochondria
Abstract
Tim8 and Tim13 are non-essential, conserved proteins of the mitochondrial intermembrane space, which are organized in a hetero-oligomeric complex. They are structurally related to Tim9 and Tim10, essential components of the import machinery for mitochondrial carrier proteins. Here we show that the TIM8-13 complex interacts with translocation intermediates of Tim23, which are partially translocated across the outer membrane but not with fully imported or assembled Tim23. The TIM8-13 complex binds to the N-terminal or intermediate domain of Tim23. It traps the incoming precursor in the intermembrane space thereby preventing retrograde translocation. The TIM8-13 complex is strictly required for import of Tim23 under conditions when a low membrane potential exists in the mitochondria. The human homologue of Tim8 is encoded by the DDP1 (deafness/dystonia peptide 1) gene, which is associated with the Mohr-Tranebjaerg syndrome (MTS), a progressive neurodegenerative disorder leading to deafness. It is demonstrated that import of human Tim23 is dependent on a high membrane potential. A mechanism to explain the pathology of MTS is discussed.
Figures
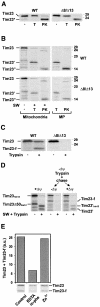
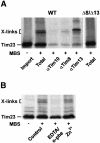
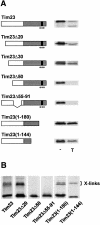
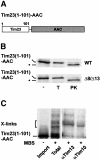
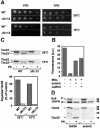
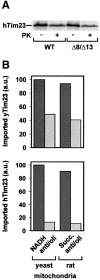
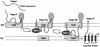
Similar articles
-
Role of the deafness dystonia peptide 1 (DDP1) in import of human Tim23 into the inner membrane of mitochondria.J Biol Chem. 2001 Oct 5;276(40):37327-34. doi: 10.1074/jbc.M105313200. Epub 2001 Aug 6. J Biol Chem. 2001. PMID: 11489896
-
The Tim8-Tim13 complex of Neurospora crassa functions in the assembly of proteins into both mitochondrial membranes.J Biol Chem. 2004 Mar 26;279(13):12396-405. doi: 10.1074/jbc.M313037200. Epub 2004 Jan 13. J Biol Chem. 2004. PMID: 14722057
-
Carrier protein import into mitochondria mediated by the intermembrane proteins Tim10/Mrs11 and Tim12/Mrs5.Nature. 1998 Feb 26;391(6670):912-5. doi: 10.1038/36136. Nature. 1998. PMID: 9495346
-
Protein import into mitochondria.IUBMB Life. 2001 Sep-Nov;52(3-5):101-12. doi: 10.1080/15216540152845894. IUBMB Life. 2001. PMID: 11798021 Review.
-
Clinical and molecular findings in a patient with a novel mutation in the deafness-dystonia peptide (DDP1) gene.Brain. 2003 Aug;126(Pt 8):1814-20. doi: 10.1093/brain/awg174. Epub 2003 Jun 4. Brain. 2003. PMID: 12805099 Review.
Cited by
-
Mitochondrial protein synthesis, import, and assembly.Genetics. 2012 Dec;192(4):1203-34. doi: 10.1534/genetics.112.141267. Genetics. 2012. PMID: 23212899 Free PMC article.
-
Toc12, a novel subunit of the intermembrane space preprotein translocon of chloroplasts.Mol Biol Cell. 2004 Nov;15(11):5130-44. doi: 10.1091/mbc.e04-05-0405. Epub 2004 Aug 18. Mol Biol Cell. 2004. PMID: 15317846 Free PMC article.
-
Novel variants of human SCaMC-3, an isoform of the ATP-Mg/P(i) mitochondrial carrier, generated by alternative splicing from 3'-flanking transposable elements.Biochem J. 2005 Aug 1;389(Pt 3):647-55. doi: 10.1042/BJ20050283. Biochem J. 2005. PMID: 15801905 Free PMC article.
-
Mitochondria use different mechanisms for transport of multispanning membrane proteins through the intermembrane space.Mol Cell Biol. 2003 Nov;23(21):7818-28. doi: 10.1128/MCB.23.21.7818-7828.2003. Mol Cell Biol. 2003. PMID: 14560025 Free PMC article.
-
Structural basis of client specificity in mitochondrial membrane-protein chaperones.Sci Adv. 2020 Dec 18;6(51):eabd0263. doi: 10.1126/sciadv.abd0263. Print 2020 Dec. Sci Adv. 2020. PMID: 33355130 Free PMC article.
References
-
- Bauer M.F., Sirrenberg,C., Neupert,W. and Brunner,M. (1996) Role of Tim23 as voltage sensor and presequence receptor in protein import into mitochondria. Cell, 87, 33–41. - PubMed
-
- Bauer M.F., Gempel,K., Reichert,A.S., Rappold,G.A., Lichtner,P., Gerbitz,K.-D., Neupert,W., Brunner,M. and Hofmann,S. (1999a) Genetic and structural characterization of the human mitochondrial inner membrane translocase. J. Mol. Biol., 289, 69–82. - PubMed
-
- Bauer M.F., Rothbauer,U., Mühlenbein,N., Smith,R.J.H., Gerbitz,K.-D., Neupert,W., Brunner,M. and Hofmann,S. (1999b) The mitochondrial TIM22 preprotein translocase is highly conserved throughout the eukaryotic kingdom. FEBS Lett., 464, 41–47. - PubMed
-
- Bauer M.F., Hofmann,S., Neupert,W. and Brunner,M. (2000) Protein translocation into mitochondria: the role of TIM complexes. Trends Cell Biol., 10, 25–31. - PubMed
Publication types
MeSH terms
Substances
LinkOut - more resources
Full Text Sources
Molecular Biology Databases